3D aerogels assembled from 2D MXenes have recently become a research focus due to their properties, performance, and ease of fabrication. A paper published in Advanced Materials has explored the creation of 3D printed MXene aerogels with enhanced electrochemical and electrical performance.
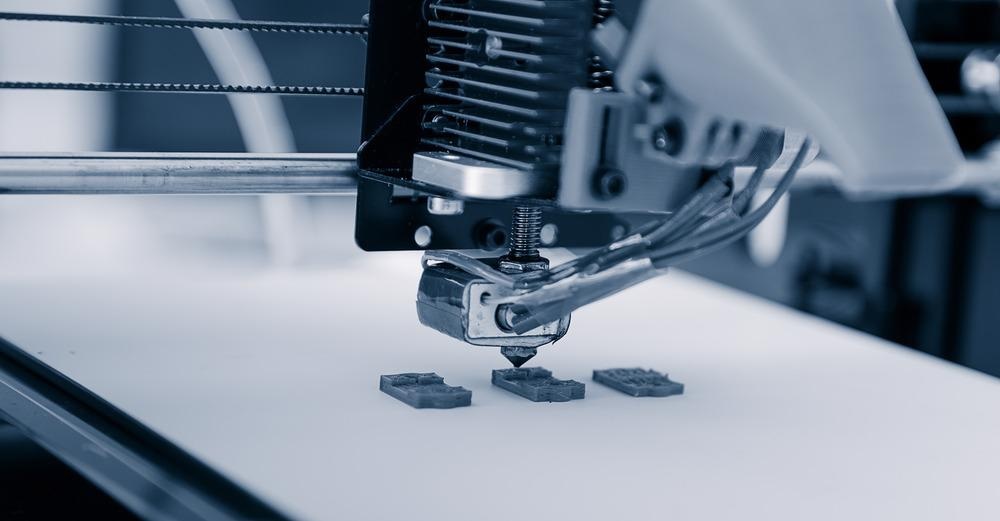
Study: 3D Printed MXene Aerogels with Truly 3D Macrostructure and Highly Engineered Microstructure for Enhanced Electrical and Electrochemical Performance. Image Credit: Alex_Traksel/Shutterstock.com
What are Aerogels?
Aerogels are 3D structures that are porous and possess low density. This class of material is the lightest solid material known to science, made up of nearly 100% air by volume. In an aerogel, gas replaces the liquid molecules within the gel without causing the gel structure to collapse.
These ultralight, solid, rigid, and dry materials are superior electrical and thermal insulators. However, they are poor radiative insulators. Common aerogel materials are silica, carbon, and metal oxides.
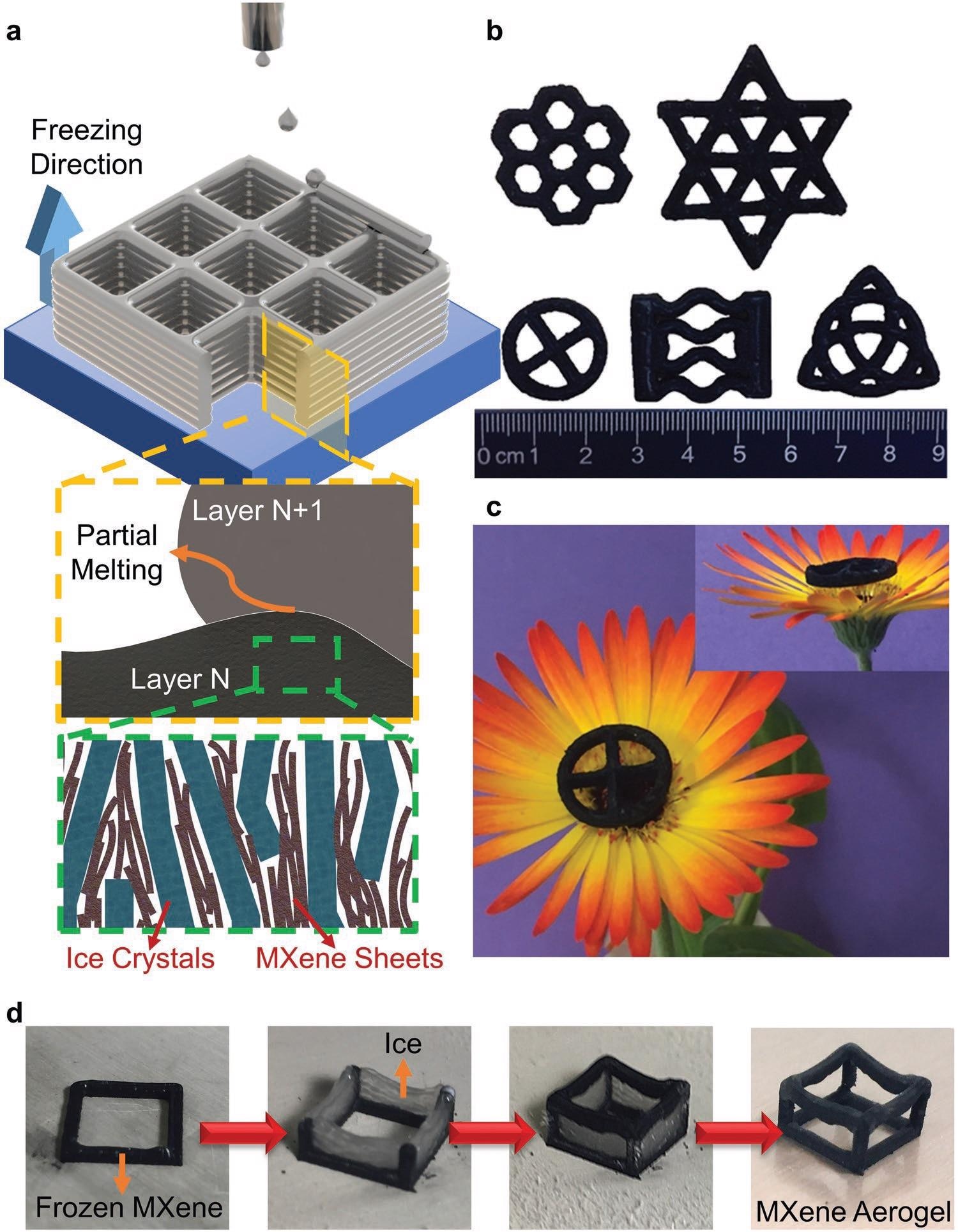
a) Schematics of the 3DFP process used for the fabrication of 3D Ti3C2Tx aerogels. MXene ink is deposited in form of spherical droplets on to a freezing substrate. Partial melting caused by the freshly deposited ink (layer N+1) ensures a good bonding of successive layers. With the freezing of the deposited ink, ice crystals grow from bottom to top, resulting in a vertically aligned porosity and MXene flakes. b) Photograph showing the 3D printed MXene aerogels having various geometries with constant cross-sections. c) Photograph showing 3D printed ultralight aerogels standing on a flower. d) Photographs showing the steps of fabricating truly 3D MXene aerogels with overhang truss structures. Image Credit: Tetik, H et al., Advanced Materials
Aerogels are structurally strong but prone to shattering under extreme pressure. The load-bearing abilities of aerogels are due to their dendritic microstructure. This structure is comprised of clusters of spherical nanoparticles (at the size range of 2-5 nm) which can be controlled during the manufacturing process. Aerogels are typically produced using a sol-gel process.
What are MXenes?
MXenes are advanced two-dimensional materials comprised of transition metal carbides, nitrides, and carbonitrides that are produced by selective etching of layers of metal atoms from their corresponding MAX phases. MXenes display enhanced properties such as hydrophilicity and superior electrical conductivity. However, much like other two-dimensional materials, MXenes have some drawbacks. They tend to aggregate and restack during assembly, limiting their performance.
To overcome these problems, numerous methods have been proposed to assemble the 2D MXenes into 3D architectures. These macroscopic structures have several useful observed properties such as a large surface area, high levels of porosity, and possess ultralight features.
The most commonly studied MXene, Ti3C2Tx, is produced by selectively etching aluminum atoms from the MXenes MAX phase. Three-dimensional structures formed from this MXene and its composites have been explored for uses such as electrochemical energy storage, water/oil separation, sensors, solar desalination processes, and electromagnetic interference shielding.
Preparing and Printing 3D MXene Aerogels
Macroscopic 3D MXene structures comprised of Ti3C2Tx have traditionally been prepared using unidirectional freeze casting (UFC) methods. A unidirectional temperature gradient is applied in this method to freeze prepared gels or solutions of the material, causing ice crystals to form on the surface. These grow along the temperature gradient, and sheets of tightly packed, highly ordered, and continuous MXenes are expelled by the ice crystals.
However, whilst this method creates 3D MXene aerogels with highly ordered microstructures, it has some drawbacks. As it is a mold-based fabrication process, it cannot create porous bodies that have complex engineered nanostructures. To overcome this issue, extrusion-based 3D printing which is then followed by UFC was proposed, which fabricated ordered honeycomb-like microporous 3D architectures with superior electrochemical performance and resistance to deformation.
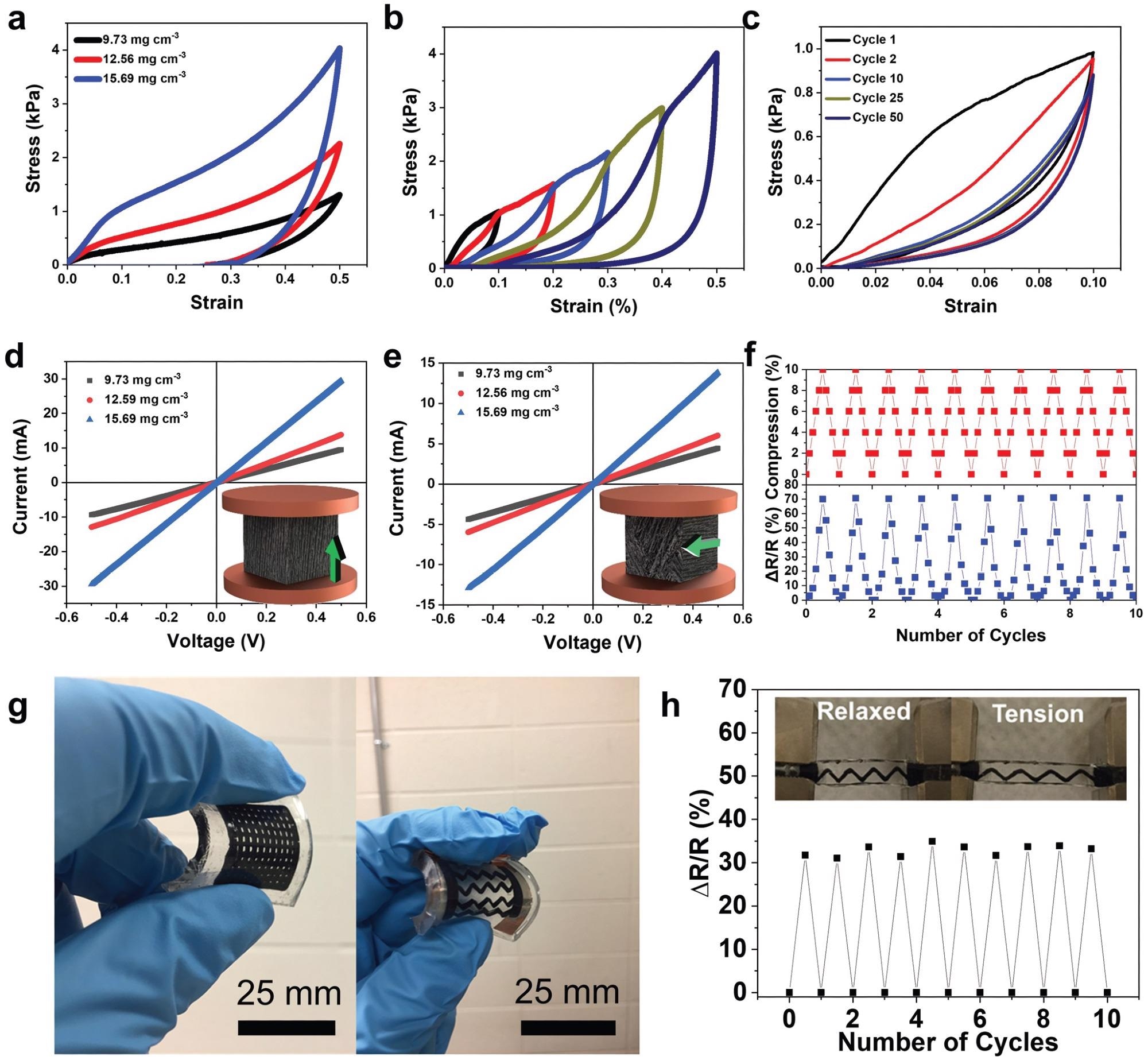
Mechanical and electrical properties of 3DFP MXene aerogels. a) Stress–strain plots of the aerogels having different densities after uniaxial compression tests up to 50% compressive strain. b) Stress–strain curves of multicycle compression by increasing strain amplitude of printed MXene aerogels (ρ = 15.69 mg cm−3). c) Stress–strain curves for 50 loading–unloading cycles up to 10% strain (ρ = 15.69 mg cm−3). d) I–V curves of Ti3C2Tx aerogels with different densities parallel to freezing direction. e) I–V curves of Ti3C2Tx aerogels with different densities perpendicular to freezing direction. The arrows in the inset of both (d) and (e) indicate the freezing direction. f) Response in the aerogels resistance to a compression with 10% strain for 10 consecutive cycles (ρ = 15.69 mg cm−3). g) 3D freeze printed MXene aerogels infiltrated in PDMS elastomer. h) Response in the resistance of the 3D freeze printed aerogels infiltrated in PDMS after applying 10% tension. Image Credit: Tetik, H et al., Advanced Materials
Although extrusion-printed MXene aerogels proved superior to their mold-fabricated counterparts, there still exist limitations in extrusion 3D printing processes. Challenges existed in fabricating truly 3D architectures with changing cross-sectional geometries and overhang features without time-consuming post-processing. Controlling the MXene sheet’s orientation was an additional challenge during processing.
Improving the Properties of 3D Printed Aerogels
The study published in Advanced Materials demonstrated a novel fabrication process termed 3D freeze printing, which combines inkjet printing and unidirectional freeze casting. By using drop-by-drop printing, the macro- and microstructures of the printed 3D MXene aerogels can be tailored. The method can use water in the form of ice as the supporting material and, unlike extrusion 3D printing, does not require a shear-thinning ink. Truly 3D structures with overhang features were fabricated.
The study characterized the printed aerogel’s electrical, mechanical, and electrochemical properties. This was used to explore their potential for use in numerous applications such as wearable electronic devices, micro-supercapacitors, and piezoresistive sensors.
The team printed all-MXene micro-supercapacitors with engineered microstructures. These had MXene sheets both horizontally and vertically aligned, demonstrating the importance of sheet alignment for improving the micro-supercapacitor device’s electrochemical performance. Vertically aligned sheets improved performance at high scan rates and provided enhanced ion transport, whereas horizontally aligned sheets improved the device’s electrical conductivity.
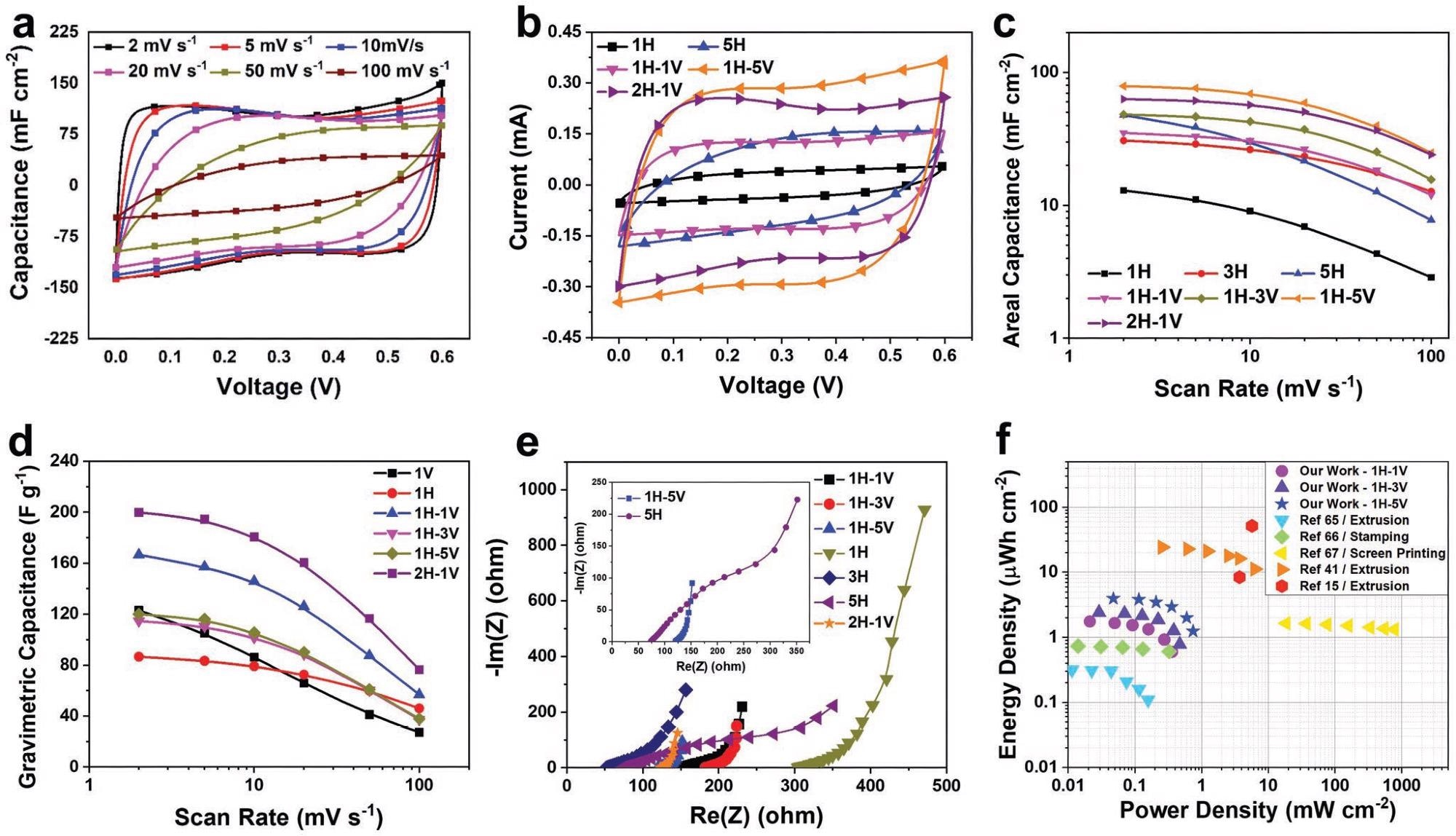
Electrochemical performance of 3DFP MXene-based MSC. a) CV at different scan rate for MSC-2H-1V. b,c) CV curves at 10 mV s−1 and areal capacitance of different 3DFP MSC devices. d) Gravimetric capacitance of MXene-based MSC compared with different MXene-based film with different thicknesses and sheets alignment. e) Nyquist plots for different MXene MSCs taken at 0 V versus the open-circuit potential. The inset shows the Nyquist plots of the MSC-5H and MSC-1H-5V. f) Ragone plots of the 3DFP Ti3C2Tx-based MSCs together with other reported values for comparison. Image Credit: Tetik, H et al., Advanced Materials
The Future
The results of the study demonstrated that 3D-printed three-dimensional MXene aerogels are attractive candidates for a slew of innovative applications in multiple industries, such as micro-supercapacitors, energy storage devices, and wearable and flexible technologies such as smart sensors. They are a low-cost, simple, customizable, and straightforward solution that can be used to fabricate electrodes with engineered, tailored micro- and macro structures.
Further Reading
Tetik, H et al. (2021) 3D Printed MXene Aerogels with Truly 3D Macrostructure and Highly Engineered Microstructure for Enhanced Electrical and Electrochemical Performance [online] Advanced Materials 2104980 | onlinelibrary.wiley.com. Available at: https://onlinelibrary.wiley.com/doi/full/10.1002/adma.202104980
Disclaimer: The views expressed here are those of the author expressed in their private capacity and do not necessarily represent the views of AZoM.com Limited T/A AZoNetwork the owner and operator of this website. This disclaimer forms part of the Terms and conditions of use of this website.