General corrosion is characterized by a uniform attack over the entire exposed surface of the metal. The severity of this type of attack can be expressed by a corrosion rate. This type of corrosion is most frequently encountered in hot reducing acid solutions.
Oxidizing agents and certain multi-valent metal ions have the ability to passivate titanium in environments where the metal may be subject to general corrosion. Many process streams, particularly H2SO4 and HCl solutions, contain enough impurities in the form of ferric, cupric ions, etc., to passivate titanium and give trouble-free service. In some cases, it may be possible to inhibit corrosion by the addition of suitable passivating agents. Anodic protection has proven to be quite effective in suppressing corrosion of titanium in many acid solutions. Almost complete passivity can be maintained at almost any acid concentration by the proper application of a small anodic potential. Table 2 gives data showing the passivation achieved in some typical environments.
Table 2. Potentials for anodic passivation of unalloyed titanium.
|
40% Sulphuric
|
2.1
|
0.2 (0.005)
|
11000x
|
37% Hydrochloric
|
1.7
|
2.7 (0.068)
|
2080x
|
60% Phosphoric
|
2.7
|
0.7 (0.018)
|
307x
|
50% Formic
|
1.4
|
3.3 (0.083)
|
70x
|
25% Oxalic
|
1.6
|
9.8 (0.250)
|
350x
|
20% Sulphamic
|
0.7
|
0.2 (0.005)
|
2710x
|
This procedure is most often employed in acid solutions having a high breakdown potential such as sulphates and phosphates. In halides and some other media, there is a danger of exceeding the breakdown potential which can result in severe pitting. The method is only effective in the area immersed in the solution. It will not prevent attack in the vapour phase.
If the use of passivating agents or anodic protection is not feasible, titanium grades 12 and 7 may solve the problem since these alloys are much more corrosion resistant than the commercially pure grades.
Crevice Corrosion
This is a localized type of attack that occurs only in tight crevices. The crevice may be the result of a structural feature such as a flange or gasket, or it may be caused by the buildup of scales or deposits. Figure 1 shows a typical example of crevice corrosion under a deposit.
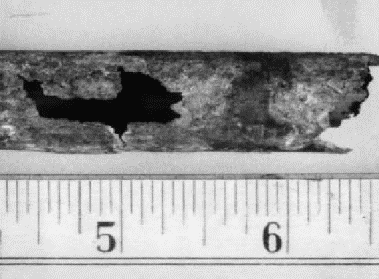
Figure 1. Crevice corrosion under a deposit
Dissolved oxygen or other oxidizing species present in the solution are depleted in restricted volume of solution in the crevice. These species are consumed faster than they can be replenished by diffusion from the bulk solution. As a result, the potential of the metal in the crevice becomes more negative than the potential of the metal exposed to the bulk solution. This sets up an electrolytic cell with the metal in the crevice acting as the anode and the metal outside the crevice acting as the cathode. Metal dissolves at the anode under the influence of the resulting current. Titanium chlorides formed in the crevice are unstable and tend to hydrolize, forming small amounts of HCl. This reaction is very slow at first, but in the very restricted volume of the crevice, it can reduce the pH of the solution to values as low as 1. This reduces the potential still further until corrosion becomes quite severe.
Although crevice corrosion of titanium is most often observed in hot chloride solutions, it has also been observed in iodide, bromide, fluoride and sulphate solutions.
The presence of small amounts of multivalent ions in the crevice of such metals as nickel, copper or molybdenum, which act as cathodic depolarizers, tends to drive the corrosion potential of the titanium in the crevice in the positive direction. This counteracts the effect of oxygen depletion and low pH and effectively prevents crevice corrosion. Gaskets impregnated with oxides of these metals have proven to be quite effective in suppressing crevice corrosion.
Alloying with elements such as nickel, molybdenum, or palladium is also an effective means of overcoming crevice corrosion problems. This is demonstrated by the performance of grade 12 and grade 7 alloys which are much more resistant to crevice corrosion than commercially pure grades.
Stress Corrosion Cracking (SCC)
This mode of corrosion is characterized by cracking under stress in certain environments. Titanium is subject to this form of corrosion in only a few environments such as red fuming nitric acid, nitrogen tetraoxide and absolute methanol. In most cases, the addition of a small amount of water will serve to passivate the titanium. Titanium is not recommended for use in these environments under anhydrous conditions. The grade 5 alloy is subject to SCC in chloride environments under some circumstances. Grades 1 and 2 appear to be immune to chloride SCC.
Anodic Breakdown Pitting
This type of corrosion is highly localized and can cause extensive damage to equipment in a very short time. Pitting occurs when the potential of the metal exceeds the breakdown potential of the protective oxide film on the titanium surface. Fortunately, the breakdown potential of titanium is very high in most environments so that this mode of failure is not common. The breakdown potential in sulphate and phosphate environments is in the 100 volt range. In chlorides it is about 8 to 10 volts, but in bromides and iodides it may be as low as 1 volt.
Increasing temperature and acidity tend to lower the breakdown potential so that under some extreme conditions the potential of the metal may equal or exceed the breakdown potential and spontaneous pitting will occur. This type of corrosion is most frequently encountered in applications where an anodic potential exceeding the breakdown potential is impressed on the metal. An example is shown in Figure 2.
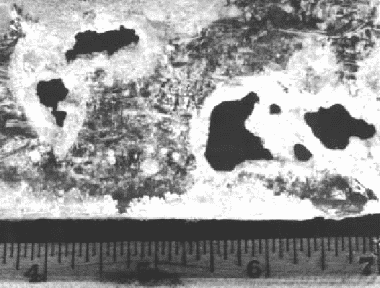
Figure 2. Anodic Breakdown Pitting of Titanium
This is a close-up view of the side plate of a titanium anode basket used in a zinc plating cell. It was a chloride electrolyte and the cell was operated at 10 volts which is about 1-2 volts above the breakdown potential for titanium in this environment. Extensive pitting completely destroyed the basket. This type of pitting is sometimes caused inadvertently by improper grounding of equipment during welding or other operations that can produce an anodic potential on the titanium.
This type of corrosion can be avoided in most instances by making certain that no impressed anodic currents approaching the breakdown potential are applied to the equipment.
Another type of pitting failure that is sometimes encountered in commercially pure titanium is shown in Figure 3.
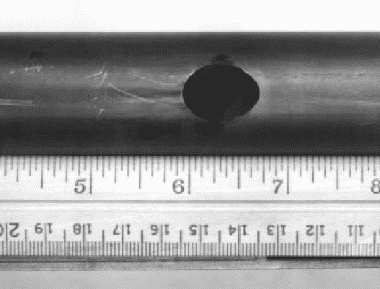
Figure 3. Unalloyed titanium tube perforated by pitting in hot brine
The specimen in Figure 3 showed scratch marks which gave indications of iron when examined with an electronprobe. It is believed the pit initiated at a point where iron had been smeared into the titanium surface until it penetrated the TiO2 protective film.
Potential measurements on mild steel and unalloyed titanium immersed in a saturated brine solution at temperatures near the boiling point gave a potential difference of nearly 0.5 volt. This is sufficient to establish an electrochemical cell in which the iron would be consumed as the anode. By the time the iron is consumed, a pit has started to grow in which acid conditions develop preventing the formation of a passive film and the reaction continues until the tube is perforated.
This type of pitting appears to be a high temperature phenomenon. It has not been known to occur below 170°F (77°C). It has not been induced on grades 7 or 12 in laboratory tests. These two alloys are believed to be highly resistant to this type of attack. However precautions should be taken with all titanium alloys to remove or avoid surface iron contamination, if the application involves temperatures in excess of 170°F (77°C). The most effective means of removing surface iron contamination is to clean the titanium surface by immersion in 35% HNO3 – 5% HF solution for two to five minutes followed by a water rinse.
Hydrogen Embrittlement
Titanium is being widely used in hydrogen-containing environments and under conditions where galvanic couples or cathodic protection systems cause hydrogen to be evolved on the surface of titanium. In most instances, no problems have been reported. However, there have been some equipment failures in which embrittlement by hydride formation was implicated.
The oxide film which covers the surface of titanium is a very effective barrier to hydrogen penetration, however, titanium can absorb hydrogen from hydrogen containing environments under some circumstances. At temperatures below 170°F (77°C) hydriding occurs so slowly that it has no practical significance, except in cases where severe tensile stresses are present. In the presence of pure anhydrous hydrogen gas at elevated temperatures and pressures, severe hydriding of titanium can be expected. Titanium is not recommended for use in pure hydrogen because of the possibility of hydriding if the oxide film is broken. Laboratory tests, however, have shown that the presence of as little as 2% moisture in hydrogen gas effectively passivates titanium so that hydrogen absorption does not occur even at pressures as high as 800 psi and temperatures to 315°F (157°C). It is believed that the moisture serves as a source of oxygen to keep the protective oxide film in a good state of repair.
Titanium is being used extensively with very few problems in oil refineries in many applications where the process streams contain hydrogen. A more serious problem occurs when cathodically impressed or galvanically induced currents generate atomic (nascent) hydrogen directly on the surface of titanium. The presence of moisture does not inhibit hydrogen absorption of this type.
Laboratory investigations and experience have demonstrated that three conditions usually exist simultaneously for hydriding of unalloyed titanium to occur:
1. The pH of the solution is less than 3 or greater than 12; the metal surface must be damaged by abrasion; or impressed potentials are more negative than -0.70V.
2. The temperature is above 170°F (77°C) or only surface hydride films will form, which experience indicates do not seriously affect the properties of the metal. Failures due to hydriding are rarely encountered below this temperature. (There is some evidence that severe tensile stresses may promote diffusion at low temperatures.)
3. There must be some mechanism for generating hydrogen. This may be a galvanic couple, cathodic protection by impressed current, corrosion of titanium, or dynamic abrasion of the surface with sufficient intensity to depress the metal potential below that required for spontaneous evolution of hydrogen.
Most of the hydriding failures of titanium that have occurred in service can be explained on this basis. Hydriding can usually be avoided by altering at least one of the three conditions listed above. Note that accelerated hydrogen absorption of titanium at very high cathodic current densities (more negative than -1.0V SCE) in ambient temperature seawater represents an exception to this rule.
Galvanic Corrosion
The coupling of titanium with dissimilar metals usually does not accelerate the corrosion of the titanium. The exception is in reducing environments where titanium does not passivate. Under these conditions, it has a potential similar to aluminium and will undergo accelerated corrosion when coupled to other more noble metals.
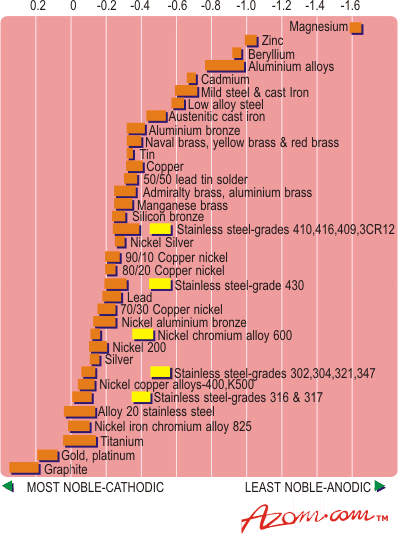
Figure 4. Galvanic series of metals.
Figure 4 gives the galvanic series for metals. In this environment, titanium is passive and exhibits a potential of about 0.0V versus a saturated calomel reference cell which places it high on the passive or noble end of the series. For most environments, titanium will be the cathodic member of any galvanic couple. It may accelerate the corrosion of the other member of the couple, but in most cases, the titanium will be unaffected. If the area of the titanium exposed is small in relation to the area of the other metal, the effect on the corrosion rate is negligible. However, if the area of the titanium (cathode) greatly exceeds the area of the other metal (anode) severe corrosion may result.
Because titanium is usually the cathodic member of any galvanic couple, hydrogen will be evolved on its surface proportional to the galvanic current flow. This may result in the formation of surface hydride films that are generally stable and cause no problems. If the temperature is above 170°F (77°C), however, hydriding can cause embrittlement.
In order to avoid problems with galvanic corrosion, it is best to construct equipment of a single metal. If this is not practical, use two metals that are close together in the galvanic series, insulate the joint or cathodically protect the less noble metal. If dissimilar metals are necessary, construct the critical parts out of titanium, since it is usually not attacked, and use large areas of the less noble metal and heavy sections to allow for increased corrosion.
|