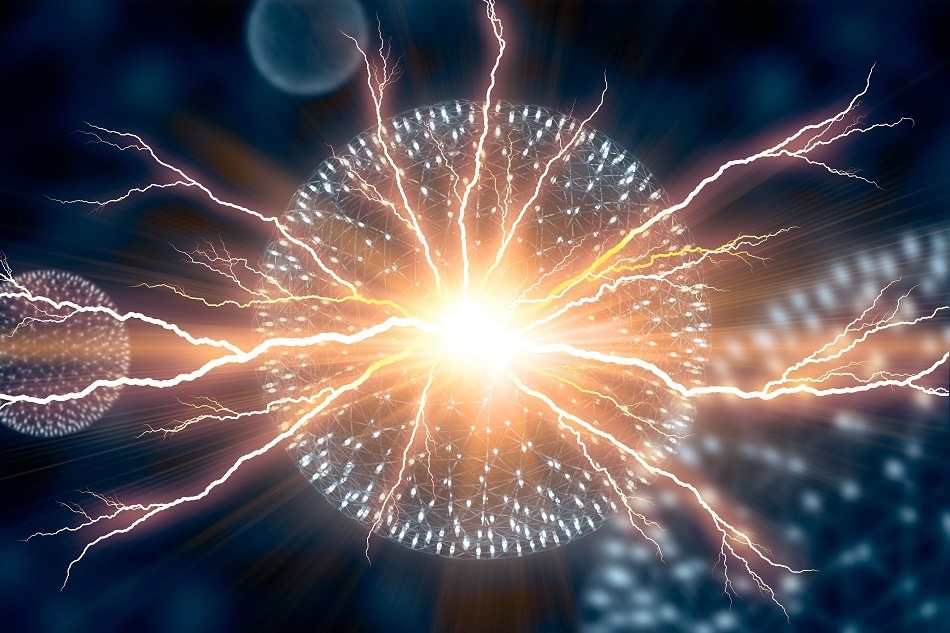
Image Credits: Quality Stock Arts/shutterstock.com
Neutron activation analysis (NAA) is a sensitive multi-elemental analytical technique, and nuclear process, that is used to determine the concentration of a range of trace elements. It is a technique that enables quantitative and qualitative information to be deduced and does so by measuring the characteristic radiation signatures—i.e. the radioactive decay—of various radionucleotides in a sample, after they have been bombarded (irradiated) with neutrons. In this article, we look at how this technique works.
NAA is an old technique which dates back decades. In its simplest form, an NAA analysis is performed by bombarding a sample—which can contain multiple elements—with slow-moving thermal neutrons, and this causes gamma rays to be emitted from the various atomic nuclei. These emissions can then be detected, and the elements can be identified precisely. Since it is an old technique, it is now used across many areas of science including chemistry, medicine, nutrition, biology, geology, archaeology, forensics, environmental monitoring, and mining.
But how that happens, and the processes that occur within the sample, are not quite as straightforward as ‘the sample just emits gamma rays’. This emission is brought about by changes in the nucleus after they have been subjected to neutrons. In NAA, the source of the neutrons is often from a nuclear reactor, but only a small amount of sample is required to perform the analysis—typically a few milligrams.
When the neutrons are released from the reactor, it collides with the atoms in the sample and a neutron gets added into the nucleus. This extra neutron addition causes the atomic nucleus in the affected element to change into a less-stable and radioactive compound nucleus. The compound then releases a gamma ray known as a ‘prompt gamma-ray’ (more about prompt gamma rays is detailed below). The nucleus then undergoes an internal rearrangement of its atomic particles, in which a β-particle is released. The nucleus then proceeds to undergo radioactive decay in the form of a gamma ray and the nucleus transforms into a stable isotope. Because each element emits a gamma ray at a specific energy and intensity, these values are cross-referenced with the known values to determine which radioisotope emitted the gamma ray, which in turn allows for the deduction of the parent atom in the sample.
One key feature of NAA methods is that they do not destroy the sample, i.e. it is a non-destructive technique, and this is the reason why they are used can be used in unconventional applications, such as for studying historical artifacts.
Prompt vs Delayed NAA
NAA techniques can be characterized in two ways and are categorized by when the gamma rays are measured, i.e. whether they are measured during the prompt gamma emission phase or when the nucleus undergoes radioactive decay. These techniques are known as prompt-gamma NAA (PGNAA or PGAA) and delayed-gamma NAA (DGNAA), respectively.
There are some differences between the two techniques. DGNAA is the conventional NAA technique as described above and measures the delayed gamma ray. DGNAA can be used with both long-lived and shorter-lived radionuclides because the neutron irradiation time can be tailored to minimize the interference in the sample—which usually occurs due to longer-lived radionuclides in the sample interfering with the shorter-lived radionuclides.
In PGAA, a beam of neutrons is often extracted from through a reactor beam port. This means that the neutron flux is of the order of 1 million times lower than in the reactor, but it means that the detectors can be placed very close to the sample. In many cases, this will offset the lack of neutron flux so that it still provides a high sensitivity. This technique is more favorable for elements with a very high neutron capture cross-sections because these elements decay too rapidly to be measured by the conventional DGNAA technique. Examples of elements which are suited to this technique include boron, cadmium, samarium, and gadolinium. Additionally, PGAA can only be used with elements that only produce stable isotopes or elements with a weak radioactive decay and gamma intensities.
Measuring the Gamma Rays
The actual measurement of the gamma rays is the most important aspect technique as it is this detection which enables the elemental composition of a sample to be deduced. So, we’re going to look at how the gamma rays are detected in a little more detail.
Overall, NAA methods can be used for studying up to 74 different elements. The detection limits towards different elements do vary, and whilst all elements can be detected in the parts per million (ppm) range, the detection limits do vary from 0.01 to 0.1 µg. The detection of elements also extends to complex media, such as human and animal tissue (as well many bodily fluids), geological deposits and rocks, and detergents, to name a few examples.
The instrumentation used to measure the gamma rays is often composed of a semiconducting detector and a multi-channel analyzer. In many cases, the detector is a hyperpure or intrinsic germanium (HPGe) detector which operates at liquid nitrogen temperatures. Therefore, the germanium crystals that make up the detectors are often mounted in a cryogenic vacuum.
Measuring gamma rays can also provide other benefits than just the elements present. NAA methods and the subsequent gamma emissions can be used to determine the concentration of different elements within a sample. This is done by simultaneously irradiating a sample of interest and a comparative standard for the specific element of interest. The irradiation of both samples is then picked up by the same detector and a series of equations can be used to back out the concentration of the element in the sample by determining the differences between the two decay signals using the half-life of the element, mass of the element, decay time and the weight of the sample and the standard.
Sources and Further Reading
Disclaimer: The views expressed here are those of the author expressed in their private capacity and do not necessarily represent the views of AZoM.com Limited T/A AZoNetwork the owner and operator of this website. This disclaimer forms part of the Terms and conditions of use of this website.