Oct 7 2020
The Abbe diffraction barrier, which fundamentally restricted the lateral optical resolution to 200 – 300 nm until the early 1990s, can be overcome by super-resolution optical fluorescence imaging techniques. These techniques were considered to be so pioneering that the 2014 Nobel Prize in Chemistry was awarded to Eric Betzig, Stefan Hell, and W. E. Moerner, “for the development of super-resolved fluorescence microscopy.”
Background
Two different techniques that are frequently used include point spread function engineering, while the other is based on single-molecule localization.
Stefan Hell’s stimulated emission depletion (STED) microscopy is associated with the first class and is allegedly a deterministic super-resolution technique. Comparatively, Eric Betzig’s photoactivated localization microscopy (PALM) is a stochastic technique.
How Does SMLM Work?
The basic principle of all SMLM implementations is the random photoactivation and subsequent localization of separate fluorescence emitters by identifying their photon emission at a specific position and time in the total absence of emission from neighboring emitters.
The centroid of the diffraction-limited distribution of photons enables determination of the location of the emitter and is much more defined than the photon distribution itself. Therefore, while a conventional widefield image is a composite of the photon distributions from a large number of emitters, a super-resolved SMLM image is a map of their individual locations that can produce lateral resolutions down to 5 nm.
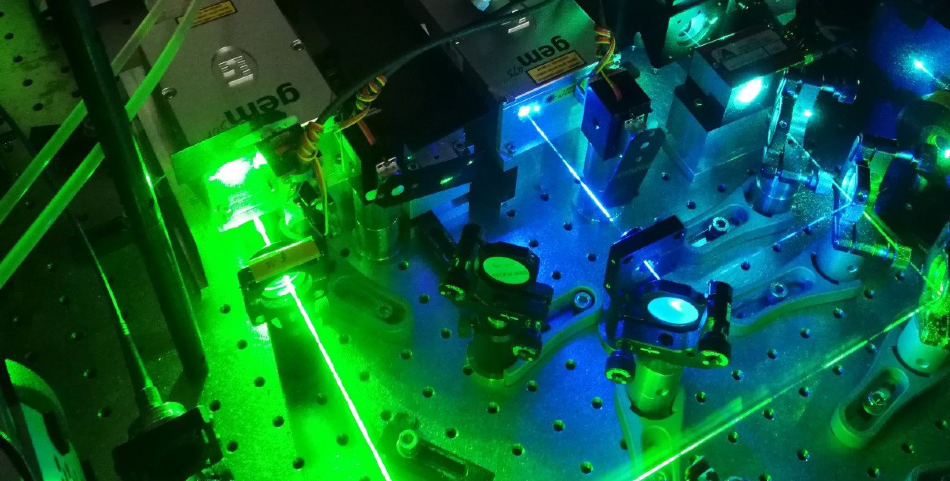
Photograph of the laser compartment of a widefield microscope for SMLM with the beam paths of the gem 473 (blue) and gem 561 (lime). Image Credit: G. U. Nienhaus and A. Kobitski (KIT)
But the question remains, how is it possible to make sure that only a small number of fluorophores emit at a specific time to make individual localization possible? By focusing on the approach used in PALM, which exploits certain properties of fluorophores that form spontaneously in photoactivatable fluorescent proteins (paFPs) of the green fluorescent protein (GFP) family, we can address the question in hand.
The main benefit of these GFP-like proteins is that they are genetically encodable so that a cell endowed with this gene generates these markers itself with no need for additional staining procedures. Generally, weakened laser light at 405 nm is selected to photoconvert a few paPFs from green- to red-fluorescent emitters throughout the acquisition of a single camera frame. Typically, Red fluorescence can be excited with a 561 nm laser. During each camera frame, the red emission emerges as a limited amount of isolated, diffraction-limited spots in the red color channel.
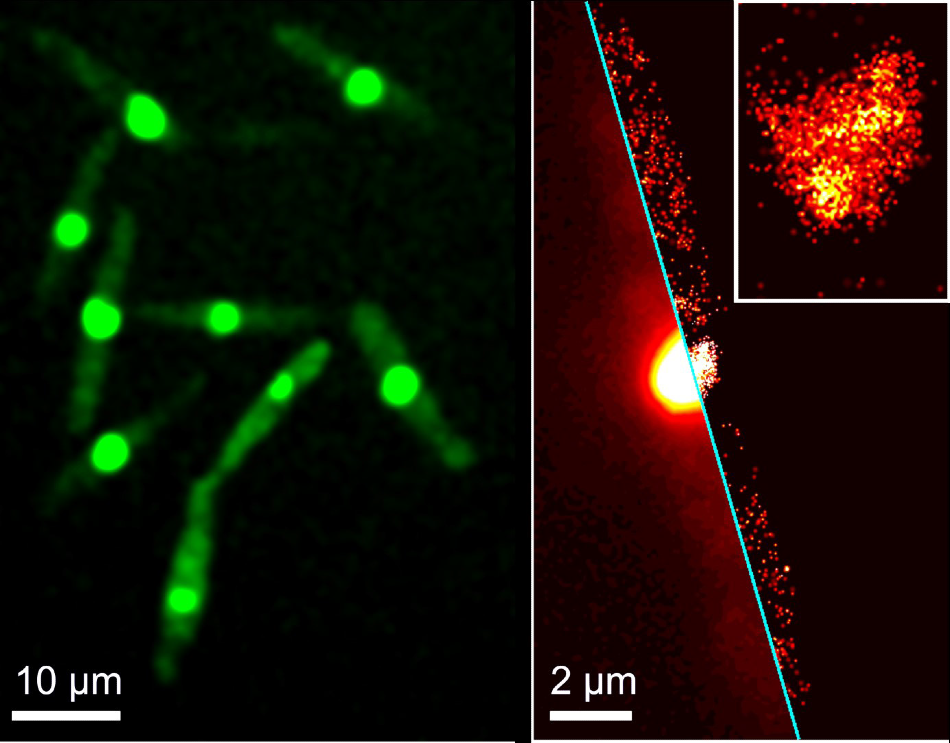
SMLM image of a selected cell with red-converted protein; the inset shows a close-up around the cell nucleus. SMLM images were rendered from 1000 successive camera frames taken with 561 nm illumination for fluorescence excitation and an additional weak 405 nm illumination for green-to-red photoconversion of the protein. Image Credit: Lu Zhou et al.: Cytoplasmic Transport Machinery of the SPF27 Homologue Num1 in Ustilago maydis, Sci. Rep. 8 (2018) 3611
Photobleaching rapidly eliminates the red-converted paFPs, so new emitters can be triggered and identified during the acquisition of the next camera frame. Cautiously modifying the experimental parameters (camera, laser powers, dwell times) reduces the probability of detecting two fluorophores with spatially overlapping intensity distributions to almost zero within a single camera frame.
One Supplier for all Wavelengths
Contrary to confocal microscopy, widefield imaging techniques necessitate illumination of a wider area with continuous high intensity. A crucial aspect in SMLM are lasers emitting at appropriate wavelengths (typically 405, 473, 561, and 640 nm) for the purpose of exciting (and possibly photoactivate) the fluorescent molecules utilized for visualization.
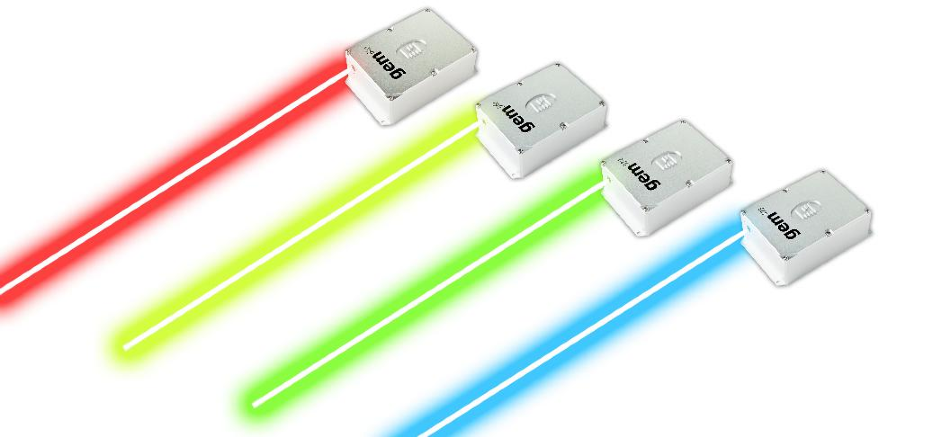
The Laser Quantum gem laser series with its 4 most popular wavelengths (473, 532, 561, and 640 nm).
High laser powers in the range of 500 mW are advantageous for fluorescence excitation of paFPs. The gem lasers from Laser Quantum provide such power and beyond at different wavelengths (473, 532, 561, 640, 660, and 671 nm) with almost perfect beam quality in a compact, cost-effective platform. The high powers also enable state-of-the-art beam shaping techniques due to the fact power is not the restricting factor as with diode lasers.
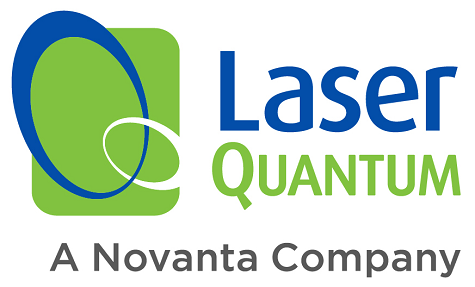
This information has been sourced, reviewed and adapted from materials provided by Laser Quantum Ltd.
For more information on this source, please visit Laser Quantum Ltd.