The demands for engineering coatings are becoming more and more stringent. Environmental concerns are also being considered as an integral part of the design process. For future economic competitiveness and a lower environmental impact we must therefore turn our attention to processes that use the minimum of resources. Thermal spraying is an attractive coating technique as it offers a wide choice of materials and processes that have a reduced impact on the environment when compared to conventional plating processes. Thermal spray coating techniques such as flame spraying, wire arc spraying and plasma spraying, allow many problems of wear, corrosion and thermal degradation to be resolved by engineering the surface with tailor-made coatings. For example, turbines can be coated by thermally spraying, allowing their use at higher temperatures. How does Thermal Spraying Work? In the thermal spraying process, heated and melted particles are propelled towards a substrate where they are flattened and quenched very rapidly. A thermal sprayed coating consists of layers of splats which have a lamellar cross section. Typically the cooling rate of each splat is somewhere in the vicinity of one million degrees per second. This rapidly quenched microstructure can have unique characteristics quite unlike those produced by conventional processing techniques e.g. the extension of solid state solubility, refinement of grain size, formation of metastable phases and a high concentration of point defects. Variations of Thermal Spraying There are many variations of thermal spraying technology. In flame spraying the combustion of a fuel gas is used to heat the material. In atmospheric plasma spraying (APS) the material is melted and accelerated in a plasma jet. To avoid oxidation of the feed material, spraying can be carried out in an inert gas atmosphere, at a reduced pressure (known as vacuum plasma spraying VPS or low pressure plasma spraying LPPS). In high velocity oxy-fuel spraying (HVOF), material is injected into a high velocity jet generated by burning a fuel mixed with oxygen at high pressure. Advantages of Thermal Spraying Compared to traditional surface modification processes, thermal spraying offers greater thickness capability, no part size restrictions, it can be performed in situ, and it produces minimal noxious waste. High processing temperatures allow deposition of many high melting point materials onto a relatively cold substrate. Applications and the Market Thermally sprayed coatings have, in recent years, gained wide spread acceptance for a variety of industrial applications - the global market for thermal sprayed coatings is currently about $1.35 billion. This growth has been primarily technology led, and high value plasma coatings hold the lion’s share of the market. A vast majority of these applications involve wear resistance, although the use of thermal sprayed coatings in combating high temperature corrosion also continues to receive considerable industrial and academic interest. These sprayed coatings are applied in order to achieve predeterminable life periods under severe operating conditions. For example, thermal sprayed coatings have been used extensively to prevent stress corrosion cracking in high strength low alloy steels used for liquid petroleum gas tanks. Other surface properties and functions that thermally sprayed coatings can provide are, biological compatibility, electrical resistance/conductivity, thermal barriers, and dimensional restoration. Extensive use of thermal spray technology has been instrumental in Europe attaining worldwide leadership in the paper and textile industry. Pioneering applications of thermally sprayed wear resistant coatings to blades, rolls and looms have allowed a significant increase in production rates. The aeronautic and space industries have also benefited considerably - many components in modern aircraft depend on hard, wear resistant coatings which can withstand temperatures of about 850°C. This type of application represents approximately 40% of the total market. Surface Protection Surface protection at high temperatures has become one of the most advanced fields of modern engineering. Diverse examples include rocket thrust chamber linings, chemical reaction vessels, combustion vanes and land-based turbines for aircraft, and hot extrusion dies. These coatings usually consist of carefully selected ceramic and metal layers which provide adequate thermal fatigue resistance and corrosion protection. The use of protective coatings for corrosion prevention has grown rapidly during the past decade, and thermal spray coatings represent a significant portion of this growth. Microelectronics Thermal sprayed coatings can also be used to manufacture hybrid microelectronic components, by spraying ceramic materials onto a metal substrate to provide electrically insulating areas. Such technology is becoming increasingly important in meeting the demands of modern computing. Biomaterials Biomaterials account for about 41% of the total weight of thermal sprayed materials in the world. Biomedical requirements in prostheses are complex and diverse in nature. Often, a combination of coating materials is needed to suit the various stages of the bio-integration process of foreign material into the human body. Functionally graded bioceramic coatings are used to enhance both the biocompatability and corrosion resistance of implants. Mechanical Properties The mechanical properties of coatings depend strongly on their microstructure and especially on the porosity and the interlamellar contacts. These depend heavily on the consumables used and the processing route used. Hardness is one of the key properties of a thermally sprayed coating, as this is often used to give a first approximation of coating wear resistance. Measurements of hardness also allow a quick estimation of coating strength and the quality of spraying, because specific processing defects, such as porosity and unmelted grains, can lower coating hardness. Wear Resistant Coatings Carbides and oxides are the hardest thermal sprayed coatings, the most commonly sprayed being WC-Co cermets. Cermet and ceramic coatings are therefore the most suitable for wear applications. The elastic moduli of the A13S sprayed coatings are significantly less than the bulk material. Carbides and oxides typically have a modulus which is 20-30% of the bulk value, though this value is heavily dependent on the processing route. Sprayed metal coatings generally have a modulus of about 30% of the bulk value, although this may be comparable with the bulk properties for coatings produced using the LLPS system. Thermal Barrier Coatings Thermal barrier coatings (TBCs) depend on low thermal conductivity and thermal diffusivity. The state-of-the-art TBC system at the moment is an air plasma sprayed Zr02/6-8wt% Y203 ceramic layer over a NiCrAlY bond coat layer, deposited by plasma spraying at low pressure. Partially stabilised zirconia has been chosen as the top coat because of its excellent thermal shock resistance, low thermal conductivity and relatively high coefficient of thermal expansion. The thermal conductivity and strain tolerance is further enhanced by the microcrack network, figure 1, produced in the individual splats due to spraying. 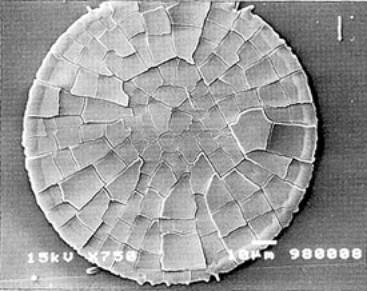 | Figure1. A typical plasma sprayed ceramic splat showing network of microcracks. | The Future In the future the manufacturing and engineering industries will continue to change dramatically, and for numerous engineering or consumer durable products appropriate surface engineering as applied by thermal spraying techniques is becoming a decisive factor in sustaining a competitive edge. Among the many surface engineering techniques available, thermal spray techniques are particularly well placed to meet the market demands. They allow the manufacture of a large variety of coatings, deposition of elaborate multimaterials (such as functionally gradient coatings) and offer a great potential for the development of new innovative coatings which can be processed with the minimal environmental disturbance. HVOF HVOF is expected to increase its share of the market significantly - hard cermet coatings are increasingly replacing conventional chrome plating, and oxidation resistant alloy coatings for turbine engines, which were sprayed exclusively by LPPS, are now also produced by HVOF. This is because the technique can produce very dense coatings with reduced oxidation and decomposition of the feed materials in air. Barrier Coatings One of the most challenging goals for thermal spray technology is barrier-type coatings for corrosion resistance, because almost complete removal of through-porosity is required. VPS has been successful to some extent, but if such coatings could be made in air, a greater number of applications could be realised. To achieve this aim, improvements are being made to APS as well as HVOF processes by introducing a gas shroud or laser skin melting. Corrosion/Wear Coatings Ceramic coatings also merit greater consideration for applications involving corrosion plus wear. However, it is important that as much care is given to selection of the bond coat as to the ceramic coating if these coatings are to perform as well as anticipated. Conclusion Another possible route for improving ceramic coatings would be to take advantage of the new wave of structural ceramics. Approaches such as fibre loading and controlled and reaction toughening could yield significant improvements in coating response. Thermal sprayed coatings may not be the answer to every problem involving surface degradation of a component, they are just one weapon in an arsenal. However, the technique does offer a great range of coating operations, and provides an appealing opportunity for innovation, while putting less pressure on environmental resources. |