Feb 11 2002
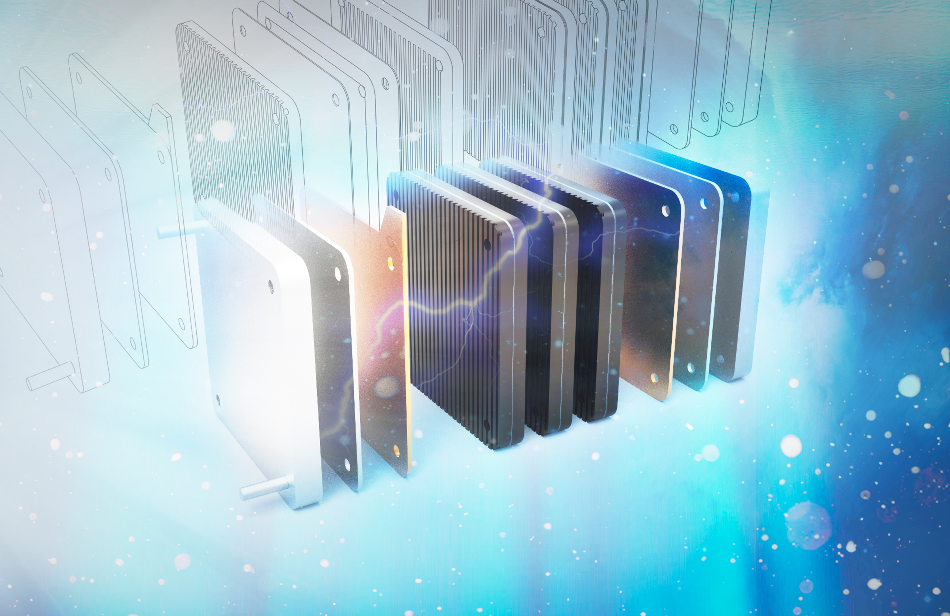
Polina Krasnikova / Shutterstock
Fuel cells are at the cutting edge of alternative fuel technology. So far, technology in the automotive industry includes platinum catalysts, and a substitute is not available even today. However, loadings are dropping quickly.
Although it took a while to develop the technology, it has now progressed well with vehicles already deployed on the road. The newest car is competitive in the small-medium car range, with respect to both performance and cost.
By 2010, the demand for automotive platinum in fuel cells should range from 500,000 to 650,000 oz. per year. As a silver lining, a fuel cell battery for cellular phones was recently introduced, which can potentially raise the levels significantly.
The Environmental Savior?
An Old Invention Now Epitomizing New Age Technology
Sir William Grove developed the first fuel cell in 1839. It took more than 120 years before the fuel cell was used in the so-called “commercial” application. That was the period when fuel cells, as with so many other cases of contemporary technology, were actively used in the NASA space program. The fuel cells were used as a compact and emission-free method for producing power. They are utilized in space even today, providing water and electricity for the space shuttle.
What is a Fuel Cell?
A fuel cell functions on the principle of hydrolysis, in which oxygen and hydrogen are mixed with the help of a catalyst to form both water and energy as a by-product. Chemists will undoubtedly reminisce wistfully about their schooldays when they come across the following chemical equation:
2H2 + O2⇒2H2O
exothermic
This is integral to the modern-day applications of fuel cells. Harmful emissions are associated with fossil-fuel technology, and the ever-increasing demand to regulate atmospheric pollutants is accelerating the search for an alternative, eco-friendly method of power generation. Many different fuel cells have been produced, or are in the process of development. The most significant of these fuel cells are briefly listed below.
The applications of fuel cells differ based on the nature of the cell. While listing all those fuel cells that are now in the public domain, the cells in which the public has shown their utmost interest to date, are those cells that are feasible for transport purposes. This would appear to be the nearest to “mass usage,” and which also involves using platinum as the catalyst.
These cells employ the proton exchange membrane (PEM) technology, utilizing a solid electrolyte. They may also be called solid polymer fuel cells. This article demonstrates how this end-application has evolved and looks at the present state of play with regard to the transport sector as a whole (see Table 1), with an aim to determine the upcoming impact on platinum demand.
Table 1. Major varieties of fuel cell
Type |
Use |
Electrolyte |
Approx Operating temperature |
Efficiency |
Comment |
Proton Exchange Membrane (PEM) |
Transport, small-scale domestic goods |
Solid Polymer |
200 °F/
80 °C |
40-50% (cf. <20% for internal combustion) |
Coming into commercial use |
Phosphoric acid (PAFC) |
Buildings, back-up generators.
Also heavy vehicles |
H3PO4 |
400 °F/
200 °C |
40-50% |
In use; 85% efficiency with cogeneration |
Molten Carbonate (MCFC) |
Large utility |
Molten Carbonate salt |
1,200 °F/
650 °C |
50-60% |
Can use unreformed fuel.
Slow delivery and response time |
Solid oxide (SOFC) |
Large, high-power utilities |
Ceramic |
1,800 °F/
1,000 °C |
45-55% |
Two 25 kW cells in use; 100 kW underway |
Alkaline |
Small scale military, aerospace |
KOH |
|
Up to 70% |
First in use; NASA program.
High purity feedstock.
Expensive |
Direct methanol |
|
Solid Polymer |
150 °F/
66 °C |
40% |
Early stages |
Regenerative |
|
|
|
|
Very early stages.
Water feedstock; recycles water by product |
Stationary Applications
Before moving on to study the PEM cells that currently appear to be the main alternative fuel source for transport vehicles, it might be worth briefly looking at other sources to gain some perspectives of where and how they could be utilized.
Falling Costs
One disadvantage with fuel cell technology is that it is still costly, albeit considerable effort is being put to reduce costs, both for non-stationary and stationary applications. Prices are inconsistent, even though it has been reported that in 1998, a power plant was offered at about US$3000/kW when compared to US$1000/kW for existing power supply systems.
Although data about the advancement of the technology is proprietary to a large extent, it was believed that this could be at least halved. It should be assumed that prices in early 2000 are en route for the projected US$1500/kW level, but the prevailing rates could not be established. In the following sections, the article looks at how the prices for automotive cells are reducing.
The PAFC Cell
By the mid-1980s, the prototype of a phosphoric acid fuel cell (PAFC) was already being used. This was the period when cells providing as much as 11 MW were installed in Japan and the United States, with a specific focus on using back-up generators in hospitals and other places. At the start of the 1990s, commercial production was ongoing, and several hundreds of cells are now being used. In particular, the Japanese are interested in developing this technology due to both space considerations and their significant reliance on oil imports.
The same considerations emphasize the aggressive work done by Japanese car firms on advancing the use of automotive fuel cells. For small-scale operators, the PAFC is the most commercially attractive fuel cell.
Alternatives
The solid oxide and molten carbonate cells take more time to build up to crucial operating rates. In addition, the molten carbonate cell can take time to react to changes in output needs. Due to their high operating temperature, these cells can operate on unreformed fuel. The solid oxide cell can use relatively impure fuels. The cells would be mainly used in utility applications for power provision.
Although the alkaline cell is the first in “commercial” use, it is one of the more exotic cells since it is extremely costly for large-scale applications. It also requires highly pure oxygen and hydrogen feedstock, albeit efforts are being made to improve its competitiveness.
The PEM Cell
The Legislative Background
The United States, specifically California, is leading the emission control legislation, with a specific focus on unburned nitrogen oxides, hydrocarbons, and carbon monoxides (all of which are now treated through PGM-containing emission control catalysts), as well as carbon dioxide (CO2, which is not treated).
The present requirements with regard to motor vehicles, as specified by the Environmental Protection Agency (EPA), involve two individual programs—the NLEV (National Low Emission Vehicles) program and the Californian LEV II (Low Emission Vehicles) program, of which the latter is the more rigorous.
The LEV program applies to California and, by choice, to Massachusetts, New York, Maine, and perhaps Vermont. Under this program, 10% of all vehicles developed as of 2003 should be Equivalent Zero-Emission Vehicles (EZEV). The emissions requirements have many complications, involving four other levels of emissions allowed in varying proportions of the car fleet; however, they may be mostly ignored for these purposes.
The Super Low Emission Vehicles (SULEVs) are an exception. It would cover those vehicles that use fuel cells driven by organic fuel and also use a reformer, as they would involve some amount of pollution.
It must be noted at this point that “vehicles” include off-road vehicles like forklift trucks and tractors for factory locations, and hence does not strictly mean 10% of passenger vehicles. (Similarly, the affinity to use fuel cells for stationary settings is the relative lack of CO2 emissions.)
What is clearly significant is that emissions legislation can only become more rigorous and that the technology rendered by fuel cells is currently at the leading edge of alternative solutions when compared to the existing technology powered by fossil fuels.
With regard to the automotive industry, battery cars provide an alternative solution, often using nickel-hydride batteries. However, these batteries need to be charged regularly, which reduces their scope of applications. Fuel cells will operate as long as there is a fuel source. While vehicles in the process of being developed could prove both popular and feasible (including those that involve both fuel cells and batteries), the performance established so far would firmly point in the direction of fuel cells as the market leader.
The relative efficiency in energy delivery between a fuel cell engine and that from an internal combustion system is shown in Table 1. A more thorough comparison is shown in the last section of the article, analyzing the specifications and the implications of the usage of platinum.
It would be sufficient to say at this point that the performance specifications, as well as the mileage range and low consumption of fuel realized between fueling, virtually sound too good to be true!
The Chemistry
Each electrode in a standard PEM cell is a carbon-based substrate covered with a platinum catalyst. At the anode, this catalyst causes hydrogen to split into electrons and protons—its two key components. Carrying a positive charge, the protons travel across the polymer electrolyte toward the cathode. On the other hand, the electrons take a different path via the external electrical circuit set up between the cathode and the anode.
Once the oxygen, electrons, and protons are in the cathode, they react together to form heat and water. When combined into a “stack,” these cells deliver adequate energy to the vehicle.
Feedstock Source
For the PEM cell, the feedstock oxygen can evidently be collected from the air. While the pure form of hydrogen can be delivered, a growing number of cell designs integrate a reformer that enables the use of ethanol, methanol, natural gas, or petroleum. Organically derived feedstock cannot be used by alkaline fuel cells because these cells produce CO2 that reacts with the catalyst. There are further alternatives to fuel cells used in a stationary setting, like gas collected from wastewater treatment or biomass plants.
Under the U.S. legislation, vehicles running from a pure hydrogen supply will qualify as zero-emission vehicles or ZEVs. Vehicles utilizing hydrocarbons can emit a small amount of CO2 and may hence need to qualify as one of the intermediary vehicles, the Super Low Emission Vehicles, or SULEVs. Although the level of emission is virtually insignificant, they may still pass the requirements for ZEVs.
Liquid Fuels
Most probably, many fuel cells onboard cars will work from a liquid fuel and include a reformer, if only because the mechanics of supplying the fuel to the vehicle are easier, and the energy density is greater from such a feedstock.
Johnson Matthey, the pioneer of this technology, received the coveted Italgas Prize at the end of the previous year for his work on producing a natural gas fuel processor called the Hotspot. The fuel processor can be used for producing hydrogen for fuel cells for both cars and small units for non-automotive domestic applications.
Hydrogen is Less Risky than has been Feared
Moreover, it has been debated that there is an unacceptable degree of risk in fuel cells carrying a hydrogen supply in an automotive vehicle. Also, the cost of converting fuel supply sources would be exorbitant. In reply to the previous point, Ford has reportedly carried out safety tests for the U.S. Department of Energy. This indicates that the technologies being developed for storing hydrogen in a fuel cell are safer when compared to those currently available for storing gasoline.
Carrying a liquid fuel and using a reformer are perhaps the most promising techniques of powering the fuel cells, but some of the comments in this article make for interesting reading:
The report states, among other things, that “a fuel cell electric vehicle would carry about 0.8GJ of hydrogen energy in a four-passenger car, or 0.2GJ per passenger… stored in fiber-composite tanks; could withstand 50-mph head-on collisions, engulfment by a diesel fire, and pressures at least 2.25 times design pressure without rupture.” Moreover, “each class of tank is also subjected to gunfire and must not explode but leak only through the bullet hole.” That was indeed some test!
In addition, the company agreed that on collision, the risk of fire (if the tank is broken) is significantly decreased because hydrogen disperses much more quickly when compared to gasoline. A fuel cell vehicle will also reduce the fire risk since it would be carrying 60% less total energy when compared to its petrol-driven counterpart.
Although liquid fuels appear to be more likely, it seems pure hydrogen—if deemed feasible—may pass several safety tests. Whether the dangers of pure hydrogen escaping into the air on impact would be “politically acceptable” is perhaps a debatable topic.
Cost Versus Environment
With respect to the delivery of fuel, be it liquid or pure hydrogen, the consultancy Arthur D. Little has projected that it would cost anywhere between US$50 and US$100 billion to offer the infrastructure for changing 10% of the U.S. car fleet to an alternative fuel. Similarly, the U.S. Department of Energy has projected that if 10% of the U.S. fleet is converted from petrol to fuel cells, oil imports can be reduced by 800,000 bbl per day. That capacity equates to nearly US$6 billion per year at an oil price of, for example, $20 per bbl (against the current level of more than $25).
Hence, the saving on imports would take approximately 10–20 years to cover the conversion cost. Based on these parameters alone, this does not appear like a commercial proposition. However, the Department of Energy also pointed out that if 10% of the U.S. fleet is converted from petrol to fuel cells, air pollutants can be reduced by one million tons per year and that the emission of 60 million tons per annum (mtpa) of CO2 can be prevented.
The power of the oil lobby should not be taken lightly. In some quarters, there is a feeling that the market share may be at risk. But the present ecological position is such that the setting may well have the upper hand—as it did in the face of protest from the oil sector in the 1970s and 1980s regarding the removal of lead in petrol to accommodate emission control catalysts. (Generally, it was not known that lead is stripped from petrol when autocatalysts are introduced as a precursor; the “lead-free” problem escalated on the back thereof.) It is of major potential importance that Exxon has decided to collaborate with Toyota and General Motors for developing fuel cell-powered cars; the consortium is investigating ways to develop cleaner petrol.
Moves Toward Fuel Cell Usage
Evidently, the jury is out as far as conversion at large scale is concerned; however, the activity of the leading car companies along with organizations like Johnson Matthey and Ballard is pioneering the way forward. Although it was initially visualized that phosphoric acid fuel cells would be feasible for automotive technology, their power density is considerably very low, and a cell that is sufficiently large to drive a car would have occupied most of the back seat.
The PEM technology is a major enhancement. The hard work by top car companies in an association, mostly, with the technology firms has currently enabled the display of prototypes at car shows.
The Major Players
The formation of dbb—a joint venture between Ballard Power Systems, Ford, and DaimlerChrysler—is one of the most recent and important developments in the evolution of the automotive fuel cell. (Moreover, Ford and DaimlerChrysler presently hold 15% and 20% of Ballard Power Systems, respectively.) The association is working well, helped by a collaboration deal signed in March 1999 with Johnson Matthey (JM). Under this agreement, JM has become dbb’s exclusive development partner for catalysts meant for hydrogen purification, together with many other applicable catalytic components.
JM already had a long-term contract with Ballard Power Systems for the mutual development and supply of catalysts, and this association promotes even closer ties between the two.
In the meantime, numerous firms are looking at advancing technology. While DaimlerChrysler is integrating fuel cells into the latest A-class model, Ballard Power Systems has currently added Nissan to its ever-increasing list of customers that already includes Volvo, Honda, and VW.
The Future
A few buses equipped with fuel cells have already hit the road. Ballard Power Systems anticipated that the streets of California would include 55 such vehicles by 2003. With respect to cars, it appears that DaimlerChrysler is the first company to venture into large-scale production. The company expected to sell a version of its NECAR in 2004, just in time to conform to the environmental legislation.
Specifications
DaimlerChrysler will display Ballard Mark 900—the new fuel cell system—to the public. The system provides 75 kW and 80 kW on methanol and hydrogen, respectively. It measures about 1 m in length, 45 cm in width, and 30 cm in height. This gives a volume of about 77 L—easily accommodating into a typical existing engine compartment.
This is about 50% the volume of the Mark 700 module, with a power density of approximately 30% higher at 0.97 kW/L on reformate and 1.04 kW/L on hydrogen. In addition, the system on which the module is based can be reconfigured for any type of car, based on power and size needs. With the module set for the manufacturing line, only the platinum loading or the nature of the cell seal may need to be changed.
Currently Viable for Small-Medium Cars
Developed for automotive applications, the standard fuel cell stack system provides 50–75 kW. DaimlerChrysler demonstrated a new system that offers 75–80 kW (see above) from a fuel cell stack. This fuel cell stack has a power density of about 1 kW for each liter, which is a significant improvement on the original designs.
Since 1 kW is approximately equal to 1.34 horsepower (hp), an 80-kW stack provides approximately 107 hp. Moreover, as the majority of the vehicles in the United States are between 60 and 200 hp, this would place them in the current systems in the small-medium range. The top of the equivalent range would need a stack of 150 kW.
Comparison with Alternative Technology
Comparing a fuel cell’s higher efficiency with that of the internal combustion engine, the consumption of fuel in a car powered by fuel cells is normally about one-third that of an equivalent petrol-powered car.
There are also other specifications that are competitive. For instance, the DaimlerChrysler NECAR 4 can achieve 90 mph, and has a range of nearly 280 miles before it needs refueling.
The car can accommodate five persons along with extra luggage space, since the fuel cell has been integrated into the vehicle’s floor. The GM EV1 and other battery-powered vehicles may need to be recharged after they cover only 90 miles. They also have very limited space (just two passengers). However, the GM EV1 is useful for short journeys and is increasingly being used in fleets of postal delivery trucks, for instance.
Platinum: Vital Component, but Loadings are Falling Fast
Platinum loading is very important. While the size of a standard fuel cell has been considerably reduced, so has its cost. However, it might need to be further reduced. In 1994, a standard fuel cell stack for a car would have cost approximately US$30,000. But by mid-1999, this was reduced to about US$500, indicating a drop by a factor of 60.
In Ballard’s 1999 Annual Information Form, the company had reported that “In 1999, the Corporation demonstrated a 75% reduction in catalyst requirements and in 1995 a further reduction of 57% was demonstrated in 1997 the Corporation began to implement the low catalyst loading technology of 1995/96 in production fuel cells.”
In 1998, the corporation showed an additional decrease of 70% in catalyst loadings. This implies that while the 1998 technology has not been implemented in the fuel cells in active use, it will be done shortly. The collective impact of such reductions is a net drop of—from the loading levels of the early 1990s—97%.
Costs
Platinum accounts for the major cost of a PEM fuel cell. In 1999, platinum averaged US$377.25/oz., indicating a loading in the latter part of the year of, maybe, an ounce or so for every stack. Newly published estimates from Johnson Matthey suggest that by the time fuel cell cars reach the large-scale production, each will require 8–10 g of platinum. Even now, the cost of platinum lies in the mid US$500–US$600 range, affected by disruptions in Russian supplies.
At the forecast level of US$420/oz. as a long-term average, the simple spot price of a platinum loading would, therefore, reduce to US$100. General Motors and Ford believe that a fuel cell-powered car of the same cost can be produced as a model, powered by a traditional internal combustion engine.
Conclusion
In 1999, 8.7 million passenger cars were sold in the United States. To infer an order of magnitude, it has been assumed that by 2010, 10% of the national fleet would be working on fuel cells and that a majority of the U.S. states will have followed California’s lead in terms of emissions.
Assuming reasonable growth in car production, but maintaining the rate of fuel cell usage at 10% only, U.S. vehicles powered by fuel cells would be consuming 280,000 to 350,000 oz. per year. With Japan and Europe following suit, this could be doubled for universal application. This would be compensated by the loss of maybe 60,000 oz. per year owing to a lack of emission control catalyst fitments.
A Half Million Ounce Market for Platinum—Minimum
This should be considered as a low case, in terms of the number of fuel cells being utilized; however, it may be high in terms of platinum catalyst loadings. Considerable advancements have already been made with respect to reducing the usage of platinum, but there is often room in these designs for more stringency.
By 2010, the fuel cell sector should be adding at least 500,000 oz. of the annual consumption of platinum to the market, with the scope for additional growth.