This article is an executive summary of a webcast titled “Polymer and Processing Challenges for the Next Endovascular Revolution,” published on October 21st, 2015, and sponsored by Zeus.
The deposition of lipids within the vessels and the subsequent formation of plaque along the vessel walls lead to arterial disease. These plaque lesions may be either soft or become hard and calcified, and as plaque accumulates, these lesions gradually reduce the luminal space within the vessel. This narrowing of the vessel interior is called stenosis. It was first treated with balloon angioplasty, which involves traversing a balloon across the vessel lesion and expanding it to push the narrowed vessel outward so as to restore blood flow.
Later, balloon angioplasty was further improved with the use of bare metal stents (BMS). In this method, the angioplasty balloon is used to make free dilatation, and then, a second balloon loaded with the metallic stent is inserted. Finally, the narrowed artery is opened up and normal blood flow is re-established by expanding the metallic stent in place.
However, with continued use of the BMS technology, several complications were also discovered. Some BMS implantations led to excessive formation of neointima within and around stent as the body tried to wall off the foreign material. The resulting hyperplasia together with the overgrowth of the new intimal layer narrows the arterial lumen and effectively reverses the effect of the BMS.
Therefore, a novel stent technology was developed to address the hyperplasia-associated vessel blockages from BMS. Drug-eluting stents, or DES, enable the release of anti-proliferative drugs from the stent after implantation. These stents were capable of reducing the hyperplasia seen with BMS and prevent vessel blockages post-implantation. In the following years, the medical sector attempted to develop a new technology that would be able to achieve an optimal combination — that is, the ability of the stent to push open the artery and deliver the drugs as required to modulate the body’s reaction to the foreign materials.
There was also a need for a methodology that would enable the vessel to return to a closer-to-normal state. This study eventually resulted in the development of bioresorbable vascular scaffold (BRS) technology. After these scaffolds are implanted arterially, they are absorbed by the body over time as they ultimately disappear.
Several limitations of previous stent therapies (Table 1) are addressed by bioresorbable scaffolds. Since balloon angioplasty also leads to acute occlusion, metal stents had been a standard procedure because of their strength and ability to preserve luminal diameter. The same benefit is provided by bioresorbable stents but they do not carry the associated hyperplasia and risk of constrictive remodeling. Until now, balloon angioplasty also did not provide the potential to deliver therapeutic agents. While this capability is provided by DES to address proliferation of new tissue, they also carry the consequences associated with implanted foreign materials.
Here too, BRS technology succeeds equally well because these bioresorbable devices are absorbed into the body and thus prevent hyperplasia in majority of cases. The degree of remodeling after stent placement or angioplasty was another area that was enhanced with BRS technology. Balloon angioplasty normally shows greater remodeling when compared to the DES or BMS techniques, but bioresorbable scaffolds support expansive remodeling because the implanted device is absorbed by the body. Since the scaffold is no longer present, normal vasomotion as well as natural stress and strain in arterial wall motion is approximated more closely, triggering natural remodeling of the arterial wall.
The Path to BRS
Percutaneous coronary intervention, also known as PCI or coronary angioplasty, is an exclusive, well-tried method that particularly targets blocked coronary arteries. Undoubtedly, a wealth of historical clinical data supports breakthroughs in PCI technology. As a case in point, a 15-year follow-up study following BMS implantation revealed that at five years, there was about 23% cumulative target lesion failure (TLF), and that at 15 years, the lesion failure rate was approximately 50% (Figure 1A). In a similar study, cumulative TLF was compared for two DES (Figure 1B). This study discovered that for Boston Scientific’s paclitaxel-eluting stent (PES) called Taxus, TLF after five years was roughly 19%. For Abbott’s everolimus-eluting stent (EES) called Xience, TLF was approximately 13% — now believed to be best-in-class.
Table 1. Potential benefits of BRS (+ Prevented or not restricted; – not prevented or restricted; NA not applicable because of absence of stent)
.jpg)
Comparing a BRS to Xience DES and Taxus, an effective BRS should show similar clinical efficacy at first but exhibit significant improvements over time (Figure 1B). After implantation, BRS is anticipated to show similar best-in-class TLF results when compared to Xience stents through approximately one year. From one to five years following implantation, BRS is predicted to exhibit a noticeable decrease in TLF as opposed to other DES, with the BRS being absorbed into the body and arterial physiological function approaching normal. If these BRS expectations are fulfilled, BRS will become the next breakthrough in endovascular therapy.
In addition to these long-term studies, numerous generations of BRS have been checked clinically for a number of years. In 2015, one-year results of the ongoing clinical trial of Absorb III (Abbott) bioresorbable vascular stent (BVS) were reported. This was a multicenter single-blind trial in which 2000 patients were given either a 2:1 ratio of Xience CoCr-EES or Absorb BVS. This prospective study included a five-year follow-up plan. The goal of the Absorb program is to show a non-inferior result with Absorb BVS as opposed to Xience EES at one year, and to show superior results of the Absorb BVS between the period of one and five years. Study results revealed that at one year, Absorb BVS was statistically non-inferior to Xience for TLF.
.jpg)
Figure 1. Clinical data showing the development path from bare metal stents to bioresorbable scaffolds. Cumulative total lesion failure (TLF) includes cardiac death, target vessel myocardial infarction, ischemia-driven target lesion revascularization.
Despite achieving non-inferiority for the Absorb BVS, wider results were in favor of the Xience EES. There was a higher level of scaffold thrombosis in the Absorb BVS study arm, which was a major concern particularly for stents implanted in smaller arteries. It was believed that the increase in scaffold thrombosis had resulted from the combination of the thick (157 μm) strut together with inappropriate or poor implantation methods. Proper implantation techniques are very important when using a thick strut scaffold: in all the cases, post-dilation with a high-pressure, non-compliant balloon is advised — a practice which is far from the prevalent DES method. With post-dilation, the scaffold is fully expanded to obtain effective strut apposition. Moreover, it is possible to further improve the overall method by embedding the strut(s) into the plaque or wall in effect, thus reducing the thickness of the strut.
Why Is Strut Thickness Important?
In addition to the possibility of restenosis linked with stent implantation, this treatment procedure also carries a risk of thrombosis. Although this potential is low, it still poses a serious threat after stent implantation. Strut thickness is a factor that has been linked to the rate of stent thrombosis. After implantation, the distal side of the stent strut has a large area of recirculation that results in a low endothelial stress domain. This low-stress domain is favorable to aggregation of platelets and thus thrombosis. This recirculation is minimized by thinner stent struts, which also help to maintain the physiologic endothelial shear stress closer to homeostatic. As a result, next-generation BRS are anticipated to be geared toward realizing stent struts that are considerably thinner.
The Ideal BRS
Realizing a perfect BRS is a multi-step process and has to be carefully considered. Among these apprehensions, the bioabsorbable tubing from which the BRS is developed is the most significant. The tubing should preferably have low leachables, toughness, strength, strength retention, thermal stability, and the potential to control the degradation of the material (Table 2). Thus, these physical properties of the bioabsorbable tubing eventually impact the attributes of the BRS itself.
Table 2. Table showing the relationship of bioabsorbable stent attributes, bioabsorbable material properties, and the stent tubing specification targets
.jpg)
With regards to the practical aspects of the BRS, a number of preferred traits come to the forefront. Among these, biocompatibility is the most important trait to date. It is primarily achieved by applying the standard range of bioabsorbable polymers — mostly polyesters. For a long time, these materials have demonstrated biocompatibility in several medical devices and also have defined, well-controlled polymer handling and extrusion procedures to prevent contamination and reduce polymer degradation. Another important factor is the good deliverability of the device. The scaffold should be able to withstand nominal and over-expansion inside the vessel, while maintaining strut apposition.
To achieve this aspect, the toughness of the tubing is enhanced through a combination of high radial strength and elongation resulting from optimal orientation of molecules. When high radial strength in the tubing is achieved, it becomes possible to reduce the thickness of the scaffold strut. For room temperature storage and to prolong shelf life, the polymer can be processed to obtain a moderate to high crystallinity. With regards to vessel support, the popular view is that scaffold or stent support should continue for a period of three months.
This period promotes proper healing and remodeling of the stent site. Strength retention is mainly modulated via the chosen base polymer and subsequently by retaining a high molecular weight or viscosity across all processing steps, leading to a tubing that has high radial strength. In addition, the selection of polymer and molecular weight controls the entire absorption timeline of BRS. Also, using polymers instead of metals comes with its own set of complications. Compared to most metals, polymers inherently have lower toughness and strength. This difference from metal stents requires careful consideration of strut design, scaffold design, and polymer processing. For instance, good device design can be undercut by poor polymer processing and vice versa. On the whole, the preferred stent material properties and stent attributes illustrated here control the specifications required for the bioabsorbable stent tubing.
Processing Challenges for BRS
A thorough understanding of materials science and polymer chemistry is not enough to achieve a clinically viable BRS. In addition, the development of these devices requires an in-depth knowledge of the handling and processing associated with the polymers. For instance, optimization of the rate of loss of mechanical integrity is considerably influenced by the polymer chemistry, but processing during the manufacturing steps also plays an important role. If there are shortfalls in either handling or processing the polymer resins, it can result in compromised absorption profiles and mechanical properties of the tubing, leading to premature loss of mechanical support and unnecessary inflammatory response.
Tubing Manufacture
The manufacturing process of bioabsorbable tubing also considers polymer chemistry with regards to morphology. For instance, when it comes to polymer selection, an ideal chemistry would be one that is rich in poly-L-lactic acid (PLLA) due to its higher glass transition temperature (Tg) and its potential to crystallize and lock in the crystallite and molecular orientation. The absorption profile of PLLA is also consistent with the critical mechanical support timeframe of three months. High-lactide copolymers such as poly(L-lactide-co-D-lactide) (PLDLA), poly(L-lactide-co-ε-caprolactone) (PLC), and poly(lactide-co-glycolide) (PLGA) are other suitable polymers for bioabsorbable tubing. Co-monomer chemistry offers the potential to modulate various properties such as strength retention, toughness, and complete absorption profiles. Therefore, careful selection of processing and polymer types to create a particular morphology offers an additional means to influence the BRS’ overall performance.
Apart from the intrinsic properties related to polymer chemistry, Zeus has refined proprietary processes for producing one of the most sophisticated BRS device components to date. The high-precision and tight tolerance extrusion techniques developed by Zeus allows the focus to be on specific properties of bioabsorbable scaffolds. These processes are management of the thermal, mechanical, and hydrolytic degradation of the polymer at the time of the handling and processing stages. This strategy also enables highly perfected morphology development in successive processing stages. Therefore, careful consideration and forethought enable the use of these specialized processing methods to achieve ideal BRS properties for each application.
Orientation processing is the final step in tubing manufacturing. This step is carried out to guide the molecular arrangement of the polymer molecules in space so as to create the required material properties. This process is widely used and is especially popular with textiles and sutures, for instance, where the ensuing material orientation is in the machine direction. However, in the case of an annular geometry, additional considerations and opportunities exist for more directed orientation. The tubing’s radial tensile strength and toughness are significantly improved by directional orientation (Figure 2). This processing step offers an extra mechanism to customize the BRS’ properties outside of any chemical considerations.
.jpg)
Figure 2. Illustration of the bioabsorbable tubing manufacturing process and the relationship of molecular orientation to strength and toughness.
Summary
Compared to previous generations of stents, bioabsorbable scaffolds present several important advantages. These types of scaffolds signify a fourth revolution in the history of stent development and are becoming popular with every new product available in the marketplace. The Absorb III BVS clinical trials showed both safety and efficacy at one year post-implantation and also stabilized acute coronary syndrome. In addition, thick struts of the first-generation bioabsorbable scaffolds underscored the significance of post-procedural sizing and implantation method. As a result, future-generation devices will concentrate on using thinner struts to deal with these concerns and to reduce the risk of other adverse events, including thrombosis.
Zeus uses a team of scientists and engineers, who have a sound knowledge of morphology development, bioabsorbable polymer chemistry, and processing methods; as a result, the company is now able to provide 100 μm wall bioabsorbable tubings that are particularly developed for BRS with good expandability, high strength, and optimal patient outcome.
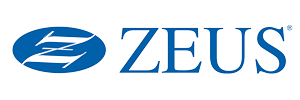
This information has been sourced, reviewed and adapted from materials provided by Zeus Industrial Products, Inc.
For more information on this source, please visit Zeus Industrial Products, Inc.