What inspired your latest research into the natural processes involved in creating complex structures?
The biggest source of inspiration was natural phenomena. Complex formations and landforms are gradually produced by long-term external stimuli like wind and water erosion, which act nonuniformly on surfaces and change with time.
Some natural formations, such as sandstone arches and pillars, marble caverns and columns, pyramid-shaped dunes and the famous Badlands Guardian, leave a strong impression of intelligent design rather than the result of a random process.
These phenomena make us wonder if we can artificially make recognizable shapes similar to the ones found in nature from nonlinear and chaotic energy fields such as the known vibration field on a Chladni plate.
How are complex natural formations such as arc-shaped rocks typically formed?
I’m not a geologist, but according to the latest theories, the emergence of these natural formations are usually extremely complex multiphysics processes, consisting of elastic deformations, frictional forces, electrochemistry, microcracks, biogenic factors, etc.
However, it’s often the external stimuli that can cause certain internal reactions and eventually drive the formed structure out of equilibrium and into a new state. From state to state, the formation is gradually shaped in an unpredictable manner.
Why have researchers never before attempted to produce these shapes by mimicking natural phenomena?
The natural phenomena of creating shapes from long term external stimuli are complex and often involve chaotic dynamics. They are also not easily predictable or replicable.
Many researchers have focused on creating a specific template - topographic features, energy wells in acoustic or magnetic fields - in the form of the desired structure or shape. These templates would allow particles or building blocks to explore the associated energy landscape and eventually be trapped at the global minimum of the energy.
Researchers often employ sophisticated hardware and processes consisting of dozens or even hundreds and thousands of transducers to produce these templates and make them reprogrammable.
In general, the relation between the template and shape is intuitive. However, the natural shape formation processes we studied here are not always that intuitive or “one-step” processes and may pass through numerous states on their way.
Can you describe how you carried out your latest research into simulating the natural processes behind these intricate shapes?
Our work was not focused on the accurate simulation of natural shaping processes but was rather inspired by them. Similar to the shape formation processes in nature directed by spatially nonlinear external fields, we used spatially nonlinear vibration fields produced on a silicon plate driven by an actuator, also known as a Chladni plate.
The vibration fields set the particles placed on the top of the plate in motion, whose direction and magnitude tend to change drastically from place to place at the same frequency and from the same areas at different frequencies.
The nature-inspired algorithm we developed iteratively selects and applies the vibration field associated with different frequencies to gradually drive the particles towards the target shape. In other words, we used a feedback loop to adjust the process to accelerate the shape-forming process in a relatively short time, instead of waiting a long time as you would in nature.
In your research, you used an algorithm to determine the most appropriate vibration frequency for the particles. Why is knowing the correct vibration frequency important, and how does the vibration frequency affect the direction of the particles?
Knowing the correct vibration frequency is important as it is that frequency that makes it possible to move the particles in the desired direction. However, knowing the correct vibration frequency is only possible when one can predict its effect on particles.
This is where machine learning came into play; we collected sufficient data based on the particle motion on locations across the plate for different frequencies and trained neural networks on that data.
Even though the motion is rather random and chaotic, the neural networks could reasonably predict the direction and magnitude of the particle motion at different locations on the plate for every vibration frequency we used.
The direction of the particles, in the long run, is towards the closest node of vibration, where the vibration magnitude is the smallest. Despite this trend, at a short timescale, the direction is uncertain and motion is chaotic.
Did you come across any challenges when carrying out your research? If so, what were they, and how did you overcome them?
Our shape formation experiments took a long time, sometimes taking many hours a day. However, most of these experiments were necessary to explore the limits of the algorithm and the hardware.
One challenge we came across was the chaotic nature of the system, i.e., particles with very similar starting points can lead to very different results.
We have the advantage of using closed-loop feedback to accelerate the shape formation process. However, the chaotic nature of the particle motion on the vibrating plate means that the results of each experiment could be quite different. On the other hand, this is very similar to the cases in nature that inspired us initially, so we are more excited than upset by such challenges.
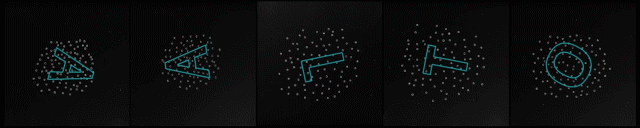
The nature-inspired algorithm selects and applies the vibration field associated with different frequencies to gradually drive the particles towards the target shape.
Was there anything that came as a surprise from the results of your research?
It came as a surprise that our algorithm had to adapt the position and orientation of the shape to the dynamic particle distribution. Otherwise, the process would often result in failure. Clearly, the resources of the vibration fields alone were not sufficient for a successful result.
Luckily, we could mathematically formulate a shape formation task as an optimization problem, very similar to the point set registration problem in computer vision in its formulation. During the shape formation process, the algorithm solved this problem iteratively and thus brought together the particles and the target shape.
This surprise raised a few interesting points: firstly, the shape-forming process in nature does not calculate the orientation or location – this is an artificial concept, so it was not a problem for our nature-inspired goal; secondly, it raised an interesting scientific question on how many degrees of freedom such a vibrating plate actually has.
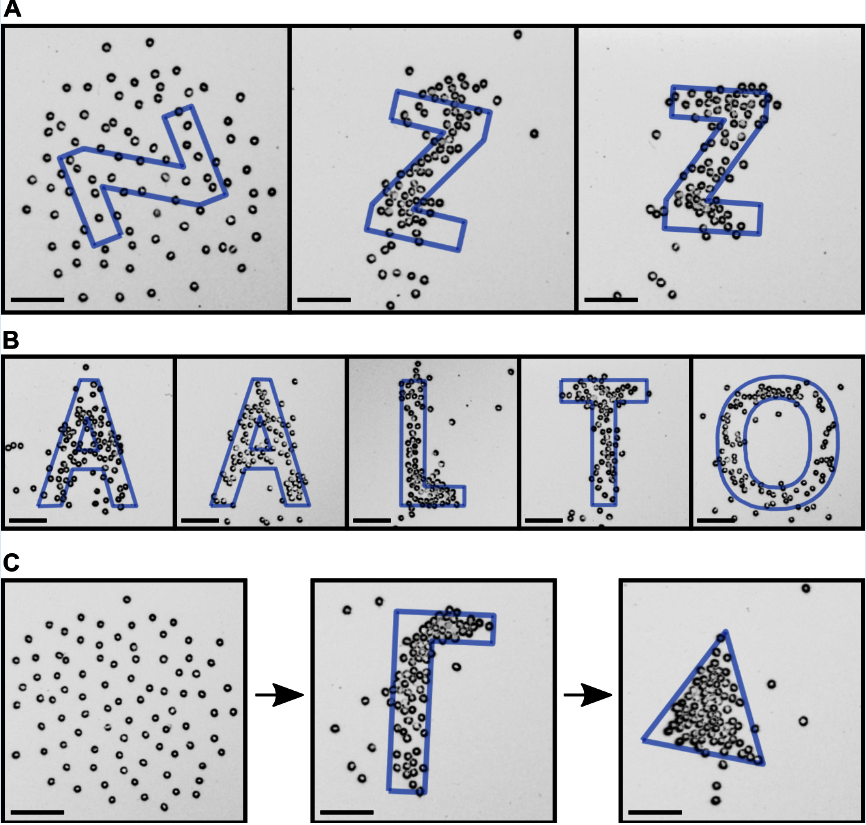
Snapshots of a shape formation experiment showing several stages of the process, from the initial particle distribution to the end result.
What are some of the future applications of your research within the materials science industry? Does your research have any applications beyond materials science?
We believe that our nature-inspired method could be used to sort cells in medical and bioscience research or may inspire novel fabrication technologies.
In addition, our approach may be applicable to other systems similar in their dynamic characteristics to a Chladni plate, such as systems with turbulent flow fields. Moving and manipulating particulate matter is essential for many industries beyond the material science industry.
What are the next steps for your research?
The next step is to substantially increase the number of particles involved in the shape formation process. We also intend on experimenting with other particle types and materials, e.g., cells or granular materials like sand. This may require us to revise our approach, both from a motion modeling point of view and from an energy field point of view.
For more information on Prof. Quan Zhou's research, visit this link.
About Prof. Quan Zhou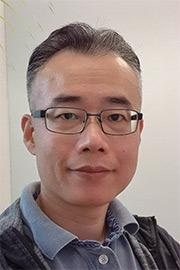
Prof. Quan Zhou received the M.Sc. degree in Control Engineering and Dr. Tech. degree in Automation Technology, both from Tampere University of Technology, Tampere, Finland. Currently, he is heading the Robotic Instrument group in the Department of Electrical Engineering and Automation, School of Electrical Engineering, Aalto University, Finland. He has over twenty years of experience in miniaturized robotics, micro- and nanomanipulation, and automation.
Prof. Zhou is currently the coordinator of the Topic Group Miniaturised Robotics of the European Robotics Association. He was the coordinator of EU FP7 project FAB2ASM. He was also the General Chair of the International Conference on Manipulation, Automation and Robotics at Small Scales, MARSS 2019, and the Chair of IEEE Finland Joint Chapter of Control System Society, Robotics and Automation Society and System Man and Cybernetics Society. Prof. Zhou has also won the 2018 Anton Paar Research Award for Instrumental Analytics and Characterization.
Disclaimer: The views expressed here are those of the interviewee and do not necessarily represent the views of AZoM.com Limited (T/A) AZoNetwork, the owner and operator of this website. This disclaimer forms part of the Terms and Conditions of use of this website.