Please can you tell the readers a little bit about yourself and your position at the Albert Crewe Centre for Electron Microscopy?
I am currently Director of the Albert Crewe Centre for Scanning Transmission Electron Microscopy at the University of Liverpool, where I lead a team of academic researchers, technical staff, PDRAs, and graduate students in the development of high spatial, temporal, and energy resolution methods for imaging both the atomic-scale structure of materials and the progression of dynamic processes controlling diffusion, energy transport, and degradation.
I have been working in this field for the last 30 years since my PhD at the University of Cambridge, and, prior to starting in Liverpool in 2017, I worked for 25 years in the US. In the US, I held appointments in academia at the University of Illinois and University of California, at several of the US Department of Energy’s National Laboratories (Oak Ridge, Lawrence Berkeley, Lawrence Livermore, and Pacific Northwest), and have formed a spin-out company based in Chicago that is pursuing commercial applications of my intellectual property in compressive sensing.
The underlying theme of my research is to develop imaging methods that will provide new insights into materials that can address society’s needs for new, clean energy generation, conversion, storage, and transmission.
How is the Albert Crewe Centre for Electron Microscopy contributing to the development of materials research?
In the ACC, we are focusing on the advancement of methods that allow the formation of realistic real-world environments in the electron microscope and to perform studies that minimize the effect of the electron beam on the sample.
While electron microscopy has provided numerous insights into materials structures over the years and aberration correction has allowed for the highest spatial resolution, most analyses being performed are with the sample in the vacuum of the microscope.
Work at the ACC aims, for example, to image materials in liquids or at atmospheric pressure so that we can understand how changes to the environment around a particular material lead to different properties. An example of this would be to study battery electrodes in a liquid electrolyte to directly image the formation of the solid electrolyte interphase (SEI) and dendrites during charge-discharge cycles.
The emphasis on dynamics means that we must take great care to use only the minimal number of electrons to form the image (electrons in the STEM/TEM can damage the sample and change the dynamics) which is why we now aim to use compressive sensing and artificial intelligence for all experiments.
The University of Liverpool has recently been awarded £4.8 million funding from the Engineering and Physical Sciences Research Council for a state-of-the-art Scanning Transmission Electron Microscope (STEM). Why is this important for the study of new materials on an atomic scale?
Many of the fundamental properties of materials are controlled by the arrangement of atoms at interfaces and defects. For many of the materials and processes being developed for clean energy applications, the diffusion of atoms at these interfaces and defects are crucial in determining the efficiency of the process, its control, degradation processes, and eventually how the material can be recycled.
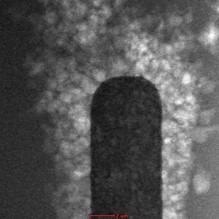
In-situ image of the deposition of Li (light contrast) on a Pt electrode (dark contrast). The tracking of the whole process in-situ allows the kinetics of the process and the key structural features to be identified. Image Credit: B. L. Mehdi, E. Nasybulin, J. Qian, C. Park, D. A. Welch, R. Faller, H. Mehta, W. A. Henderson, W. Xu, C. M.Wang, J. E. Evans, J. -G. Zhang, K. T. Mueller, and N. D. Browning, Nano Letters 15, 2168-2173 (2015)
By studying structures and processes on the atomic scale, we can assess both the fundamental limit of a particular property and the mechanism to control/improve it for any application.
To follow on from the battery example above, our goal in looking at battery systems is to understand which parts of the interface between the electrode and electrolyte control the solvation/desolvation of Li-ions during charge/discharge cycles.
Can you tell us how a Scanning Transmission Electron Microscope works and how it compares to a Transmission Electron Microscope (TEM)?
Both the STEM and TEM use electrons that are transmitted through the sample to form an image, which requires both techniques to use very thin samples (typically less than 100 nm thick). The differences between the methods relate to the way the beam is projected onto the surface of the specimen and the features of the scattering that are used to form the image.
In the case of the TEM, the electron lenses are used to make a large area plane wave illumination on the specimen, and atomic-resolution images are formed by phase-contrast – differences in the phases of the scattered electron wave across the specimen lead to an interference pattern that has the features of atoms.
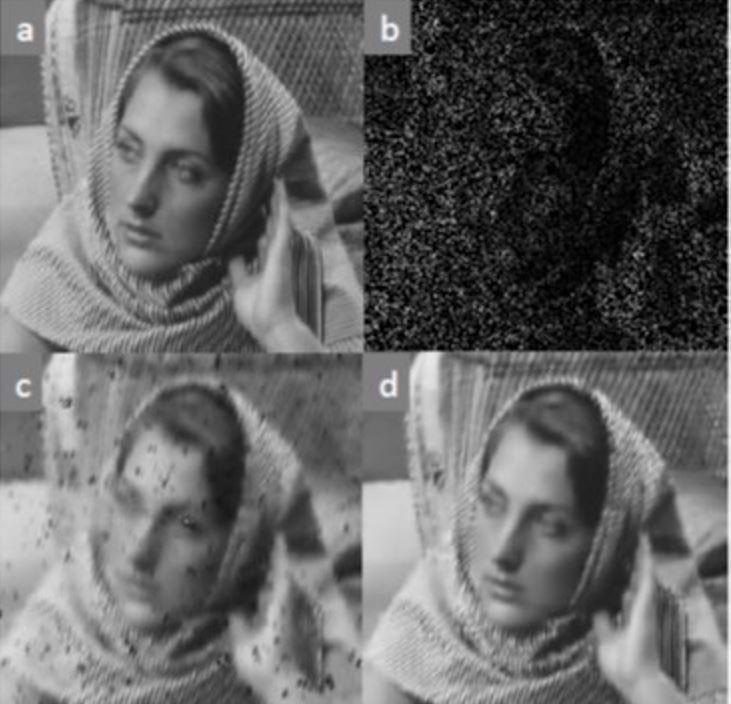
(a) Public domain test image, 'Barbara'. (b) 25% randomly subsampled image of 'Barbara'. (c) The missing information can be recovered using an inpainting algorithm, which when optimised gives a good representation of the original image. This inpainting method can be used to deliberate sample experiments at lower sampling rates (reducing damage) and then the missing information can be recovered. Image Credit: D. Nicholls, J. Lee, H. Amari, A. J. Stevens, B. L. Mehdi, N. D. Browning, Nanoscale 12, 21248-21254 (2020).
In the STEM, the lenses are focused down to as small a spot as possible (typically ~0.1 nm, in atomic dimensions) and this spot is rastered across the specimen while detectors collect the scattering at each point.
In the most common high angle detector, contrast approximates to Rutherford scattering and the image can have the same atomic spatial resolution as the beam size.
The new STEM being used by the University of Liverpool is developed with cutting-edge artificial intelligence (AI). How will this be a key factor in transforming the microscope’s capabilities?
Electron microscopes have had the ability to see individual atoms/columns of atoms for 50 years now. The development of aberration correctors in the 1990s led to increases in signal levels that mean that it is now routine to be able to see individual atoms.
However, the electron beam itself causes damage to the specimen while the image is being formed – essentially changing it during the experiment. This means that there is a large range of new materials – organics, hybrids, nanomaterials – where the beam causes too much damage for them to be imaged on the atomic scale.
The new microscope uses the latest advances in compressive sensing (i.e., sampling less of the sample) and machine learning (i.e., inpainting the missing information) to lower the dose below the damage threshold. These methods open pathways for imaging whole new classes of materials, and dynamics in every class of materials, where the results are free from beam-induced alterations.
How has the STEM microscope revolutionized materials characterization and allowed researchers to move forward with the development of advanced energy technologies and biomaterials?
The ability to see the structures of energy systems, organics, and biomaterials on the atomic scale is the key to understanding functionality.
In the case of energy systems, STEM has led to an understanding of the structure of anode/cathode materials and the kinetics of lithium intercalation for next-generation Li-ion batteries. Work on biomaterials in STEM is a much newer field and here the field is still pushing the boundaries (with new microscopes such as the one coming to Liverpool) to get to that point.
Are there any notable research findings that would not have been possible without the use of a STEM?
One of the most important areas being developed in the last few years has been the use of in-situ stages to create liquid/gas environments around the samples. These new environments have led to a range of research results investigating the fundamentals of nucleation and growth, biomaterials in native environments, self-assembly of organics, and direct operando observations of battery and catalyst functions.
How does electron microscopy challenge the limits of spatial, temporal and energy resolution?
There is only one experimental method that can directly image individual atoms distributed within/on a sample, and that is electron microscopy. In addition to aberration correctors making atomic-resolution routine, advances in detectors and pulsed electron sources mean that the temporal resolution of observations can follow the natural diffusion pathways of atoms or look at the fundamental timescale of electronic/magnetic interactions or the making/breaking of bonds.
Another recent development with monochromators has resulted in spectroscopy being performed with the same sensitivity as IR spectroscopy. Individual molecules can be identified on surfaces and interactions can be probed with the atomic-scale spatial resolution of the microscope.
How does the STEM allow observations and quantification of diffusion processes in new classes of transformative materials? What does this mean for the future of materials science?
By immersing the sample into its native environment (gas or liquid), being able to bias the sample electrically, optically, or thermally, or being able to apply a stress/strain to the sample, we can now see atoms moving when the material is under the same conditions as it would be when it functions.
Previously, microscopy would have to be performed before a stress/strain/optical/chemical test was performed and then after the ex-situ test, the sample would be cut a prepared for STEM. The goal of the microscopist would then be to try and identify changes that occurred and correlate them with an understanding of the process that took place.
By seeing the process directly, there are many more insights into multi-step dynamics that can be obtained. This can rapidly lead to the development of new structures, compositions, and architectures that will enhance the performance of energy technologies, as well as many others.
Are you able to talk about any new developments in higher spatial and temporal imaging/spectroscopic methods?
In addition to the new STEM, we are also developing a new Relativistic Ultrafast Electron Diffraction and Imaging (RUEDI) facility that will push the temporal resolution of observations to the femtosecond level. The facility, which is being developed as part of the UKRI infrastructure program, will be a collaboration between the University of Liverpool and other partners, and the microscope will be based at Daresbury Laboratory. Coupled with the new STEM in the ACC and the UK SuperSTEM at Daresbury, the Liverpool City Region will be home to the most advanced electron microscopy techniques in the world.
Energy storage systems are becoming even more important in society. How is the development of STEM solutions helping to advance this industry further?
Optimizing the energy density, lifetime, and recyclability of energy storage systems is key to the UK moving towards a carbon-neutral transportation and energy economy. The STEM is accelerating the ability to understand how each combination of electrodes/electrolytes functions and can rapidly test degradation mechanisms and options for recycling. Accelerating the materials selection, interface design, and overall life cycle of battery systems through these detailed atomic resolution experiments is a key part of the STEM methodology.
Have there been any challenges when developing STEMs and how have these been addressed?
There are always unforeseen challenges in developing new instrumentation. These challenges range from the room stability that the microscope is housed in, the control of the environment in the microscope column, the physics of the scattering process, and the quality of the images.
All developments in STEM wherever they have been performed have been the results of intensive effort to track down the limitations and create the best imaging system. Perhaps the biggest of these challenges was in the development of aberration correctors that unlocked much of the power of the STEM/TEM that we see today. There, it was a case of computer speed and electronic stability catching up with the physics for aberration correction.
Do you know of any future developments in relation to STEM that are significant to the future of materials science?
As stated above, the control of the beam artifacts during imaging will be key to the implementation of STEM methods to a wider range of materials systems. STEM is not used for organics or hybrid materials in the same way as it is for semiconductors and ceramics, and the use of AI will address this issue.
Do you have any important research that you will be carrying out in the future that you are able to discuss?
Although we know in principle how to use AI to improve the image quality and analytics for imaging in the microscope, the methods are currently too slow to be used routinely. We are currently working on increased speed and the use of machine learning to increase the autonomy of state-of-the-art microscopes – essentially, we are looking to create the world’s first self-driving STEM.
Where can readers find more information?
Readers can learn more about the Albert Crewe Centre for Electron Microscopy by visiting our website: https://www.liverpool.ac.uk/albert-crewe-centre/
About Professor Nigel Browning
Professor Nigel Browning is currently the Chair of Electron Microscopy in the School of Engineering and the School of Physical Sciences and Director of the Albert Crewe Centre for Electron Microscopy at the University of Liverpool (since 2017).
He received his undergraduate degree in Physics from the University of Reading, U. K. (1988) and his Ph. D. in Physics from the University of Cambridge, U. K (1991). He has held positions at Oak Ridge National Laboratory (1992-1995), Lawrence Berkeley National Laboratory (2003-2006), Lawrence Livermore National Laboratory (2006-2011), and most recently was a Laboratory Fellow and Initiative Lead for the Chemical Imaging Initiative (CII) at the Pacific Northwest National Laboratory (PNNL) (2011-2017).
Nigel Browning was also Assistant/Associate Professor of Physics at the University of Illinois at Chicago (1996-2002) and Professor of Materials Science (2003-2011) and Professor of Molecular Biology (2009-2011) at the University of California-Davis.
He has over 30 years of experience in the development of new methods in electron microscopy for high spatial, temporal, and spectroscopic resolution analysis of engineering and biological structures. He is a Fellow of the American Association for the Advancement of Science (AAAS) and the Microscopy Society of America (MSA).
Browning received the Burton Award from the Microscopy Society of America in 2002 and the Coble Award from the American Ceramic Society in 2003 for the development of atomic resolution methods in scanning transmission electron microscopy (STEM). With his collaborators at LLNL, he also received R&D 100 and Nano 50 Awards in 2008, and a Microscopy Today Innovation Award in 2010 for the development of the dynamic transmission electron microscope (DTEM).
He has over 400 publications (~30,000 citations, h-index=89) and has given over 300 invited presentations on the development and application of advanced TEM methods.
During his career, he has been the primary research advisor for over 50 PhD students and Postdoctoral Research Associates, many of whom now lead their own research programs at institutions around the world.
Most of his research career has been spent in the US, where he was involved in 57 research projects supported by federal and local government, non-profit foundations, and industry. In these projects, he was directly responsible for $25M, out of the $190M total funding for all the projects, including leadership of the $42M/7-year CII at PNNL.
For the past four years, he has been working as a co-founder and Vice-President for Strategy at a start-up company (Nuxutra) that is commercializing intellectual property he co-developed during his time as a researcher at PNNL (10 patents in total related to compressive sensing, super-resolution, and machine learning for advanced imaging technologies).
In the UK, Professor Browning’s main area of research has been related Li-ion batteries, being a collaborator on the Faraday Institution supported Recycling (ReLiB) project and the Principal Investigator on the Faraday Institution supported Characterisation project. In the case of the recycling project, his work has primarily been focused on understanding the chemical and biological recycling kinetics, with a view to developing new methodologies that can be incorporated into the UK economy.
Disclaimer: The views expressed here are those of the interviewee and do not necessarily represent the views of AZoM.com Limited (T/A) AZoNetwork, the owner and operator of this website. This disclaimer forms part of the Terms and Conditions of use of this website.