New research conducted by scientists at Duke University has demonstrated how the use of chalcogenide glasses could be developed into a wider range of applications, including underwater communications, environmental monitoring, and biological imaging applications. While continued research is required, the initial results are promising, and developments may be reported rapidly over the coming years.
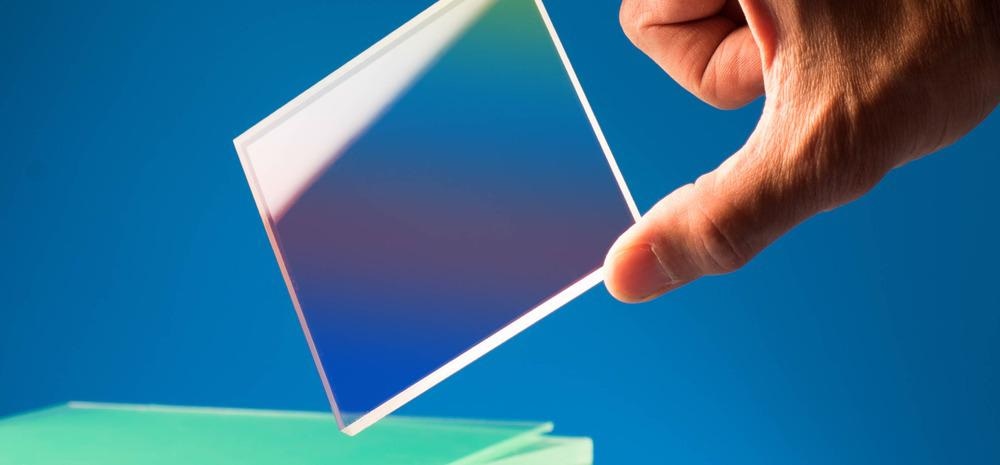
Image Credit: luchschenF/Shutterstock.com
The Promise of Chalcogenide Glasses
Chalcogenides are formed from one or more chalcogens, such as sulfur, selenium, and tellurium. They exist in a range of different colors, which are determined by their chemical composition. They have very different properties in comparison with silica glass, which is generally a passive material, whereas chalcogenides have active properties and are highly nonlinear.
Chalcogenide fibers have been demonstrated to transmit light at wavelengths beyond what is possible with silica. Additionally, chalcogenides are unique because they are photosensitive; while this makes them an inappropriate material for some applications, it opens up the door to the development of many other applications. Finally, chalcogenides are also semiconductors, which gives them useful electrical properties.
Due to their advantageous properties, chalcogenides have been leveraged into advanced electronic applications, including optical switching, molecular fingerprinting, and ultra-small direct laser writing. They are already widely used commercially in detectors, lenses, and optical fibers. They have also been proven useful for medical applications such as laser surgery.
However, because they are able to strongly absorb visible and ultraviolet wavelengths of light, the photonic applications of chalcogenide glasses have been limited to those within the near- and mid-infrared wavelengths.
For many years, chalcogenides have been used in the near- and mid-infrared wavelengths, but have been limited in their applications in visible and ultraviolet wavelengths. Now, engineers at Duke University, North Carolina, have revealed how nanostructures impact the way chalcogenides respond to light, which may offer a route to overcoming these limitations.
Generating and Transmitting the Original Frequency and at its Third Harmonic
In a study published in October 2021 in the journal Nature Communications, the team of electrical engineers at Duke describe how altering the physical shape of chalcogenide glasses extends their use into parts of the electromagnetic spectrum that had previously remained out of scope for this material.
In previous theoretical research, it had been suggested that the semiconductor that is commonly used in electronic applications, gallium arsenide (GaAs), might respond to light in a way that is unique to the behavior of its bulk and thin-film counterparts.
Due to the interactions of high-intensity optical pulses with the nanostructured form of GaAs, composed of thin wires of the material stacked together, it was predicted that it may be possible to generate higher-order harmonic frequencies (shorter wavelengths).
More on Chalcogenide Glasses - Thermal Properties of Chalcogenide Glass After Addition of Silver
In an investigation that set out to test this theory, researchers deposited a 300-nanometer-thin film of arsenic trisulfide atop a glass substrate. Then, using a combination of electron beam lithography and reactive ion etching, the material was nanostructured into arsenic trisulfide nanowires measuring 430 nanometers in width and spaced 625 nanometers apart.
While arsenic trisulfide absorbs light with wavelengths above 600 THz (around the color cyan), the team found their the nanowires were transmitting signals at 846 THz (wavelengths that comfortably sit within the ultraviolet spectrum).
By illuminating the nanowire metasurface with near-infrared light, the material generated and transmitted light at both the original frequency and at its third harmonic. These findings were rather unique, given that the third harmonic wavelength falls within the range that should be absorbed by the material.
The team’s findings demonstrated a counterintuitive result. It was theorized that it was produced as a result of the production of the nonlinear third harmonic "phase locking" with the original frequency. This is where the initial wavelength traps the third harmonic produced, enabling them to both pass through the material, preventing the third harmonic from being absorbed.
Future Directions for Chalcogenide Research
The team plan to conduct further studies to investigate whether the engineering of different chalcogenide shapes might carry the third harmonic more efficiently than the nanostrips used in the initial study. They theorize that assembling the chalcogenides in long, thin, blocks intermittently spaced at certain distances may produce a stronger signal at the third and second harmonic frequencies.
The team also hypothesizes that stacking layers of metasurfaces might also enhance the effect.
Following these continued research projects, if successful, the team could potentially establish a method of using chalcogenides in a range of visible and ultraviolet applications for both electronic materials and mid-infrared photonic materials.
After some research and development, it is believed that this work will result in establishing applications for chalcogenide glasses in underwater communications, environmental monitoring, and biological imaging. As these studies are conducted, even more potential applications of chalcogenide glasses may emerge. The potential of this field is unknown.
References and Further Reading:
Anscombe, N., 2011. The promise of chalcogenides. Nature Photonics, 5(8), pp.474–474. https://www.nature.com/articles/nphoton.2011.155
Gao, J. et al., 2021. Near-infrared to ultra-violet frequency conversion in chalcogenide metasurfaces. Nature Communications, 12(1). https://www.nature.com/articles/s41467-021-26094-1
Ken Kingery. (2021). Smuggling light through opaque materials. [Online]. Phys.org. Available at: https://phys.org/news/2021-10-smuggling-opaque-materials.html (Accessed 25 October 2021)
Disclaimer: The views expressed here are those of the author expressed in their private capacity and do not necessarily represent the views of AZoM.com Limited T/A AZoNetwork the owner and operator of this website. This disclaimer forms part of the Terms and conditions of use of this website.