Lithium-ion batteries have long reigned supreme in an era where energy storage plays a pivotal role in powering our rapidly advancing world. However, the quest for more sustainable and efficient energy solutions has driven scientists and researchers to explore alternative electrode materials that could revolutionize the battery industry.
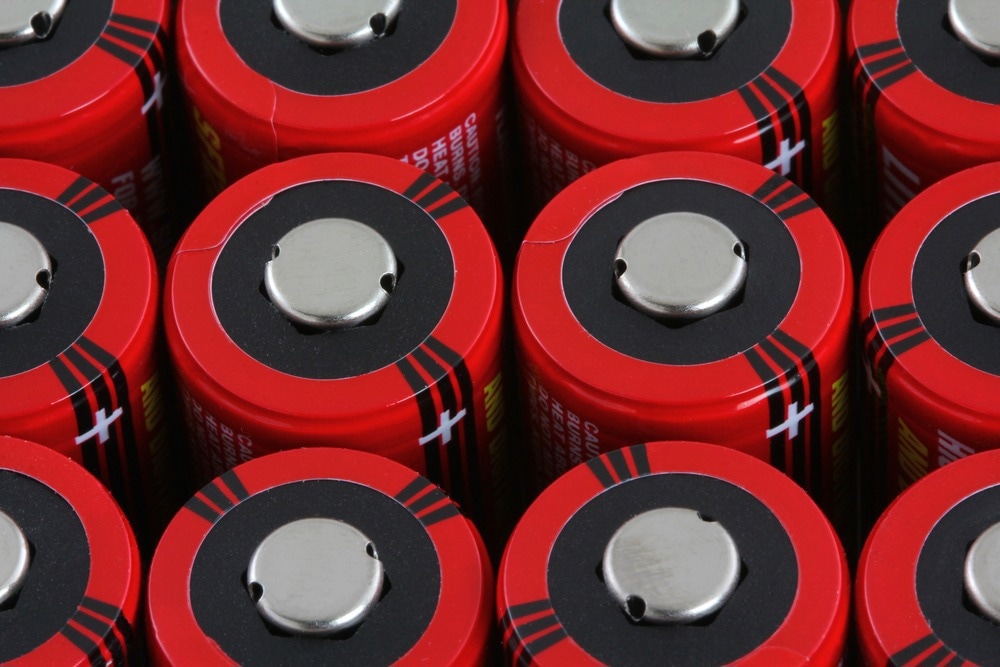
Image Credit: sasimoto/Shutterstock.com
In this article, we discuss alternative electrode materials for high-performance batteries, examining their advantages and disadvantages compared to lithium.
What is the Commonly Used Battery Electrode Material and Its Challenges?
Lithium-ion batteries dominate the rechargeable battery market due to their high energy density, long cycle life, and low self-discharge. However, the mining and processing of lithium, a key component in Lithium-ion batteries, pose environmental challenges due to its scarcity. This has contributed to a growing interest in exploring alternative electrode materials for batteries.
In addition, these novel batteries will be expected not only to outperform their lithium counterparts but also to address the concerns surrounding scarcity and environmental harm.
Researchers prioritize sustainability and are considering abundant and non-toxic elements like sodium and potassium for low-cost and eco-friendly batteries, such as sodium-ion batteries. We now discuss these alternatives to lithium as electrode materials for high-performance batteries, including their various strengths and weaknesses.
Sodium-ion Batteries
With similarities to lithium, sodium presents a promising alternative for electrode materials. Sodium-ion batteries utilize sodium ions instead of lithium ions, offering several advantages. Sodium is more abundant and widely available than lithium, leading to lower costs and reduced dependency on scarce resources.
Sodium-ion batteries also possess higher thermal stability, reducing the risk of overheating and potential safety concerns associated with lithium-ion batteries. However, the larger size of sodium ions results in slower diffusion kinetics, limiting the overall energy density of sodium-ion batteries.
Aluminum-ion Batteries
Aluminum is an appealing alternative to lithium due to its abundance and low cost. Aluminum-ion batteries show great promise, particularly for large-scale applications such as grid storage. The trivalent nature of aluminum ions allows for higher charge storage capacity compared to monovalent lithium ions.
Moreover, aluminum batteries can be fabricated using environmentally friendly materials, minimizing the ecological footprint. However, the challenge lies in finding suitable electrolytes and cathode materials that can effectively accommodate aluminum ions, as the trivalent charge requires different chemical properties compared to lithium.
Magnesium-ion Batteries
Magnesium, abundant and widely distributed in the Earth's crust, has captured the attention of researchers exploring alternative electrode materials. Magnesium-ion batteries offer the potential for higher energy density and better safety compared to lithium-ion batteries. Magnesium ions also possess a bivalent charge, allowing for increased charge storage capacity.
However, the main hurdle in developing practical magnesium-ion batteries lies in the limited number of suitable electrolytes and cathode materials that can efficiently handle the reversible magnesium intercalation and deintercalation processes, hindering the battery's overall performance.
Potassium-ion Batteries
Potassium is another element gaining recognition as a viable alternative electrode material. Potassium-ion batteries present advantages similar to sodium-ion batteries, including cost-effectiveness and reduced reliance on scarce resources. Potassium-ion batteries have also shown promising potential in achieving high energy density, outperforming traditional lithium-ion batteries.
However, like sodium, the larger size of potassium ions poses challenges in terms of diffusion kinetics, limiting the overall power density of the battery.
Zinc-ion Batteries
Zinc is a promising alternative to lithium as an electrode material in batteries. It is abundant, more stable, and lower cost. Zinc-ion batteries have high energy density, high power density, and long cycle life. In addition, they could be safer than lithium-ion batteries because they do not have the risk of thermal runaway.
However, efforts to make zinc commercially viable at scale have been few and far between. Generally, zinc-ion batteries suffer from low Coulombic efficiency, leading to capacity fading over time. They also suffer from dendrite formation, which can cause short circuits.
Calcium-ion Batteries
Calcium-ion batteries utilize calcium ions as the charge carrier instead of lithium ions. Calcium is abundant and inexpensive, making it an attractive alternative. Calcium-ion batteries have the potential for high energy density and improved safety compared to lithium-ion batteries. The challenge remains in finding suitable electrolytes and cathode materials that can efficiently accommodate calcium ions.
Metal-Sulfur Batteries
Metal-sulfur batteries combine a metal (such as lithium, sodium, or magnesium) with a sulfur-based cathode. Metal-sulfur batteries offer high energy density potential and can utilize abundant and low-cost materials. However, like lithium-sulfur batteries, metal-sulfur batteries face challenges related to sulfur's low electrical conductivity and the formation of intermediate polysulfide compounds.
Vanadium Redox Flow Batteries
Vanadium redox flow batteries utilize the flow of vanadium ions in an electrolyte solution as the charge carrier. Vanadium redox flows are well-suited for large-scale energy storage applications due to their long cycle life and high scalability. They offer advantages such as high energy efficiency, quick response times, and independent control of power and energy capacity.
Vanadium redox flow has the disadvantage of lower energy density compared to lithium-ion batteries, making them more suitable for stationary applications rather than portable devices.
Conclusion
Energy plays a critical role in our modern society, and the need to ensure sustainability along the entire value chain of products storing energy is essential. Lithium is widely used as electrode material in modern batteries but falls short in terms of sustainability and supply.
Efforts to address these limitations have included exploring alternative electrode materials that will perform better, have high availability, and be more environmentally sustainable.
Unconventional alternative electrode materials made of sodium-ion, aluminum-ion, magnesium-ion, potassium-ion, etc. could bring lasting solutions. With unique advantages and daunting challenges, these alternatives promise a vibrant, unpredictable future fueled by innovation and the pursuit of eco-friendly power.
More from AZoM: How do Semiconductors Contribute to the Development of Microprocessors and Microcontrollers?
References and Further Reading
Ali, U. (2019). Beyond lithium: alternative materials for the battery boom. Power Technology. URL https://www.power-technology.com/features/lithium-battery-alternatives/ (accessed 6.21.23).
Lourenssen, K., Williams, J., Ahmadpour, F., Clemmer, R., Tasnim, S. (2019). Vanadium redox flow batteries: A comprehensive review. Journal of Energy Storage 25, 100844. https://doi.org/10.1016/j.est.2019.100844
Utmel Electronics (2022). Lithium-ion Battery: Structure, Working Principle and Package [Online]. Utmel.com. URL https://www.utmel.com/blog/categories/battery/lithium-ion-battery-structure-working-principle-and-package (accessed 6.21.23).
Xu, H., Chen, H., Gao, C, (2021). Advanced Graphene Materials for Sodium/Potassium/Aluminum-Ion Batteries. ACS Materials Letters. 3, 1221–1237. https://doi.org/10.1021/acsmaterialslett.1c00280
Zhu, N., Zhang, K., Wu, F., Bai, Y., Wu, C. (2021). Ionic Liquid-Based Electrolytes for Aluminum/Magnesium/Sodium-Ion Batteries. Energy Material Advances 2021. https://doi.org/10.34133/2021/9204217
Disclaimer: The views expressed here are those of the author expressed in their private capacity and do not necessarily represent the views of AZoM.com Limited T/A AZoNetwork the owner and operator of this website. This disclaimer forms part of the Terms and conditions of use of this website.