The production method of a superconductor influences its usefulness. Metallurgy, which involves understanding the structure and processing of superconductors, plays a vital role in determining their electrical conductivity. This article is intended to provide an overview of the metallurgy of superconducting properties and cites specific examples from recent studies.
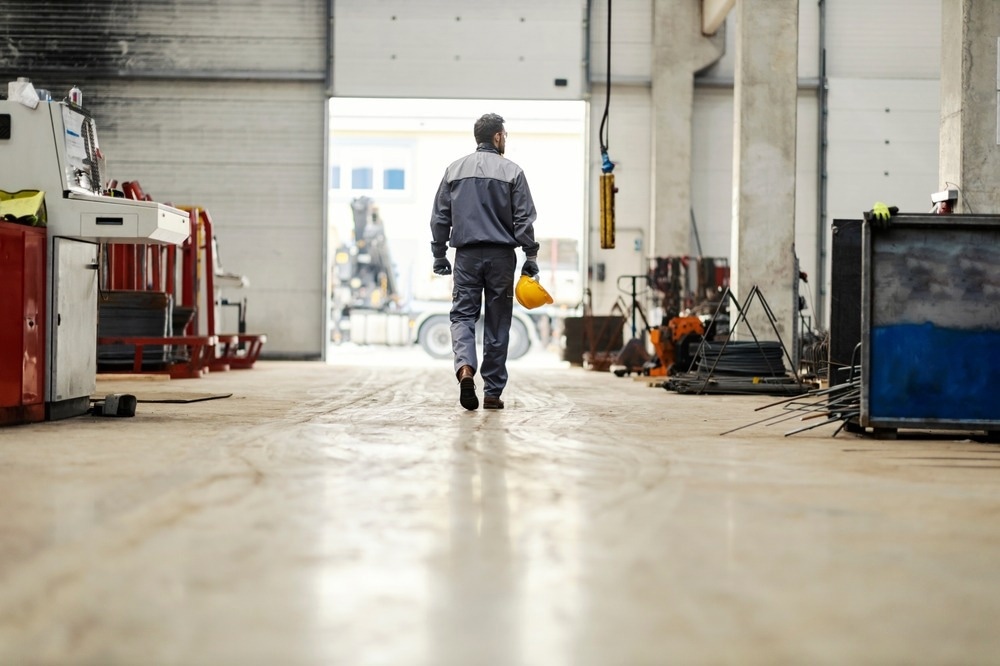
Image Credit: Dusan Petkovic/Shutterstock.com
Superconductors and Their Base Materials
During electrical conduction, resistance leads to energy loss. However, at a critical temperature, superconductors conduct electricity with zero resistance; that is, they conduct direct electricity without energy loss.
Superconductivity is a fascinating quantum phenomenon that was first observed in mercury cooled to the temperature of liquid helium, which is approximately -452 °F and only a few degrees above absolute zero. Researchers have since observed superconductivity in other materials, such as various metals and niobium-titanium alloys, which can be easily shaped into wires.
The discovery of superconducting wires presents a new challenge for researchers because these wires have zero electrical resistance and can support high electrical currents. However, if the current exceeds a specific "critical current," the electron pairs break apart, and the superconductivity abruptly ends.
The emergence of superconducting wires has led to a wide range of novel applications of this material. For example, these wires can be wound into coils to form magnets. In the 1970s, scientists utilized superconducting magnets to generate the high magnetic fields required for magnetic resonance imaging (MRI) machines. Recently, researchers incorporated superconducting magnets into synchrotrons and accelerators at scientific user facilities to guide electron beams.
Metallurgy of Superconducting Materials
Superconducting materials, particularly those designed to generate high magnetic fields, possess a critical surface in a three-dimensional (3D) system that is affected by the temperature, transport current density, and external magnetic field.
The critical temperature and fields are mainly determined by the homogeneous composition phase of the material, which can be either an equilibrium or metastable phase. The microstructure has a minimal impact because the superconducting coherence length is typically smaller than dimensions such as the grain size.
However, microstructural features, such as dislocation networks, grain size, and precipitates, have a significant effect on the critical current density. Therefore, two distinct types of metallurgy come into play, with the first addressing the problems of phase stability and the second focusing on defect structures.
The behavior of many superconductors, particularly those with high critical temperatures, can change significantly depending on how they are prepared and treated with heat. To comprehend or anticipate these variations, it is crucial to examine the specifics of their alloy phase diagrams.
Recent Studies
A study published in the Journal of Alloys and Metallurgical Systems highlighted the discovery of new quinary body-centered cubic (BCC) high-entropy alloy (HEA) superconductors. The valence electron concentrations (VECs) of these supercapacitors were in the range of 4.6 to 5.0.
The results of the study showed that these alloys demonstrated the fascinating behavior of separating into two different BCC phases with slightly varying chemical compositions as the VEC increased. This phenomenon can be attributed to the enthalpy of the formation of each binary compound.
The alloys that were examined were all categorized as type-II superconductors, with their superconducting critical temperatures varying from 3.25 K to 4.38 K. To further analyze their properties, the Vickers microhardness, which showed a positive correlation with the Debye temperature, was measured, and compared to the microhardness values of other bcc HEA superconductors.
This study demonstrated that the critical temperature consistently decreases as the hardness increases beyond a specific threshold of approximately 350 HV. Additionally, the critical temperature was plotted against VEC for quinary bcc HEAs, displaying an asymmetric VEC dependence.
The relationship between hardness and critical temperature, as well as the asymmetrical critical temperature dependence on VEC, was attributed to the combined influence of the electron-phonon coupling and the electronic density of states at the Fermi level.
Another study published in the Journal of Alloys and Metallurgical Systems utilized three melt-refining processes for 440 stainless steel, namely, silicon reduction (440 M-Si), aluminum reduction (440 M-Al), and electro-slag remelting (440 C-ESR).
The findings revealed that after undergoing three distinct melt refining processes, the metal primary carbides present in 440 stainless steel were M7C3 and M23C6. The carbides present in 440 M-Al and 440 M-Si were extremely fine particles, whereas the carbide found in 440 C-ESR was a small block.
The 440 M-Al carbides consisted of both micron and nanoscale particles. These carbides solidified and aggregated at the unit cell boundary, resulting in a primarily chromium carbide (Cr7C3) structure. The carbide particles exhibited gaps between them, and the solid-solution carbon content of the adjacent matrix was high. This made the hardness of the matrix greater than that of the carbide aggregation area.
In contrast, the matrix of 440 C-ESR dissolved many alloying elements, and the fine lump carbide was primarily Cr7C3. The carbide diffusion interface layer of 440 C-ESR improved the bonding characteristics of the interface between the carbide and matrix, consequently enhancing the mechanical properties.
Conclusion
Overall, the process of producing superconducting materials has a significant influence on their practical application, and the field of metallurgy plays a crucial role in understanding and improving their properties. By studying these materials, which can conduct electricity without resistance, researchers have opened up new possibilities for a wide range of technological applications.
The metallurgy of superconducting materials, particularly those designed for producing high magnetic fields, involves careful examination of the critical temperatures, transport current density, and external magnetic fields. The composition phase of a material, whether in the equilibrium or metastable state, determines the critical temperature and fields.
The relationship between the critical temperature and hardness, as well as the asymmetric dependence on the valence electron concentration, highlights the combined influence of electron-phonon coupling and the electronic density of states.
Furthermore, microstructural features, such as dislocation networks, grain size, and precipitates, affect the critical current density, leading to two distinct metallurgical considerations addressing phase stability and defect structures.
Recent studies have emphasized the need for a thorough understanding of metallurgical principles in the development and optimization of superconducting materials for various applications. As technological advancements continue, exploring metallurgical aspects will undoubtedly contribute to unlocking the full potential of superconductors in various fields, from medical imaging to high-energy physics.
More from AZoM: Machine Learning Approaches for Accelerated Materials Discovery
References and Further Reading
Kuo, Y. A et al. (2023). A study on structural characteristics and metallurgical mechanism of carbides for 440 martensitic stainless steel with different melt refining processes. Journal of Alloys and Metallurgical Systems, 3. https://doi.org/10.1016/j.jalmes.2023.100029
Hattori, T. et al. (2023). Metallurgy, superconductivity, and hardness of a new high-entropy alloy superconductor Ti-Hf-Nb-Ta-Re. Journal of Alloys and Metallurgical Systems, 3. https://doi.org/10.1016/j.jalmes.2023.100020
Metallurgy and Superconductivity Accessed on 13 January 2024 https://www.sciencedirect.com/science/article/pii/S2949917823000202
Disclaimer: The views expressed here are those of the author expressed in their private capacity and do not necessarily represent the views of AZoM.com Limited T/A AZoNetwork the owner and operator of this website. This disclaimer forms part of the Terms and conditions of use of this website.