Processing Techniques Most composite applications can be divided into three categories determined by performance requirements as defined by reinforcement characterisation: • Structural: continuous aligned fibres • Semi structural: continuous non aligned fibres • Non structural: discontinuous fibres Table 1 outlines the main areas of composite consumption in the USA in the period 1991 to 1994. While the majority of the industries identified in table 1 cover more than one application type, they can be generally classified into one of the above. The major processing routes used to fabricate components within these performance bands are represented in figure 1, together with the annual production quantities normally associated with these processes. It is apparent that there is a lack of process technology which addresses the volume markets in the structural and semi structural areas. This factor is a major contributor to the limited growth of composites in the industries requiring such components. Table 1. Composite shipments for the period 1991-1994 from the USA in millions of kg. | Aerospace | 17.6 | 14.7 | 11.5 | 11.0 | Business equipment | 61.3 | 65.0 | 66.9 | 72.9 | Construction | 190.5 | 219.1 | 240.4 | 270.7 | Consumer products | 67.4 | 73.6 | 75.2 | 79.3 | Corrosion resistant products | 161.0 | 150.7 | 159.7 | 170.7 | Electrical | 104.8 | 117.9 | 124.7 | 135.8 | Marine | 124.7 | 138.1 | 144.8 | 164.9 | Transportation | 309.4 | 340.2 | 372.9 | 428.9 | Other | 33.5 | 37.8 | 40.5 | 46.2 | Total | 1070.2 | 1157.1 | 1236.6 | 1380.4 | 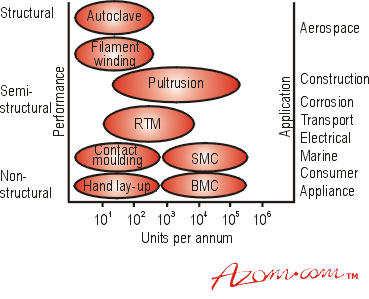 | Figure 1. Composite manufacturing processes. | Productivity Productivity increases are already being attained by the combined efforts of raw material suppliers, equipment manufacturers, trade moulders and end users. Target productivities for four processing techniques are shown in figure 2. Processing techniques can also be classified in terms of open mould processes (e.g. hand lay up, filament winding), closed mould processes (vacuum bag moulding, RTM, SMC) and continuous processes (pultrusion). 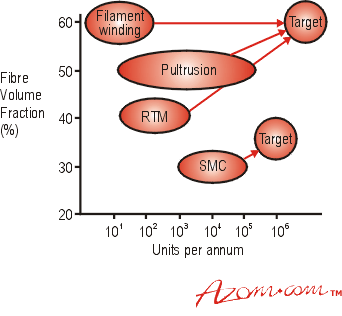 | Figure 2. Target productivities for composite manufacturing processes. | Sheet Moulding Compounds Until the mid 1980s the moulding of Sheet Moulding Compounds (SMCs) was considered a slow process, but nevertheless capable of delivering both small and large non-structural components with a surface finish superior to all other composite processing methods. SMC can refer to both the material and the process. Complex shapes and details that may not be possible with sheet metal can often be achieved relatively easily with SMC. The material usually comprises a filled polyester resin and glass fibre, which is either chopped, continuous, or a mix of both. When ready to mould, it normally has a consistency similar to that of thick putty, as it will be partially cured (B staged). As the name suggests, SMC is supplied in sheet form and in many respects can be considered a low performance prepreg. The sheets are essentially compression moulded to final shape. Cycle Times Commonly achieved cycle times were of the order of four minutes, resulting in processors operating multiple dies and ancillary equipment to keep up with production demands from their main customer, the automotive industry. Whilst this increases cost, it also enhances further the risk of process or part variations. A major deficiency of SMC components has been their structural inconsistency caused by uneven distribution of reinforcement during flow of the charge material. Much effort has been focused on the SMC moulding process in recent years, which has led to the 1 part per minute barrier being challenged. A key development enabling this improvement has been vacuum assisted moulding. Vacuum Assisted Moulding In conventional SMC processes, charge loading and press closure speeds are selected with the primary aim of forcing out entrapped air. Typically, `stacks’ of charge are placed in the mould, covering only 40% of the mould surface, and mould closure rates are in the order of 0.1 m.min-1. With vacuum assistance, a one atmosphere vacuum is applied prior to closing the mould. This enables the charge to be spread to cover up to 90% of the mould surface and closure speeds at 0.9 m.min-1 to be achieved without entrapping air. The increase in mould surface coverage by the charge has several additional benefits associated with the reduced materials flow. Wave patterns and flow lines are eliminated and localised strength of components is enhanced due to better retention of fibre orientation. Specially introduced orientation is now feasible and a high strength SMC process is available where unidirectional fibres are arranged at specific locations. Raw material suppliers are developing faster reacting resin systems designed to take advantage of the lower flow, higher speed requirements. Equipment manufacturers are investing in automation and control of the process. These continuing activities are positioning SMC for a resurgence of interest in high volume semi structural applications. Pultrusion In the pultrusion process (figure 3) dry reinforcements are impregnated with a specially prepared low viscosity liquid resin system and drawn through a die heated to about 120-150°C where curing occurs. The solid laminate, which has assumed the shape of the die, is withdrawn by a series of haul off grippers and cut to length or coiled. Pultrusion is unique among the processes under consideration in that it is capable of producing complex components on a continuous basis. The process can basically produce any shape that can be extruded. It is also not allied to any one industry and applications range from civil engineering to electrical. These factors combine to give pultrusion one of the highest predicted growth rates of all composite process. 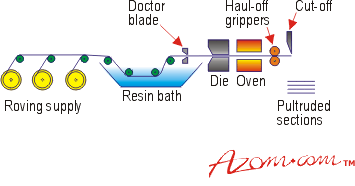 | Figure 3. Schematic of the pultrusion process. | Currently, the requirements of pultruders and their suppliers are stronger market development and higher productivity to capitalise on these projections. Faster production rates are one way of achieving this, but the industry itself has recognised the need for greater consistency of raw materials and process operation, coupled with the ability to monitor the product quality more closely. Polyester-Based Resin Systems At present, production is dominated by polyester-based resin systems, which have relatively wide processing windows. This has resulted in pultrusion remaining primarily an art rather than a science. Over the last five years equipment manufacturers have been concentrating on the provision of more sophisticated machinery, with the ability to run multiple tools. The industry has been striving to develop monitoring and control systems which permit the process to run more efficiently by reducing scrap levels and decreasing commissioning time normally associated with new dies or materials. A better understanding of the process has enabled more challenging resin system to be considered and ongoing programmes include the evaluation of phenolics and thermoplastics. Phenolic Resin Systems Traditional phenolic materials pose three main problems to the pultrusion process: volatile release, high reactivity and corrosion. These result in high void contents, narrow processing windows and rapid tool wear. Process modifications have been implemented and new resins formulated to allow more `user friendly’ and reproducible processing. Phenolic pultruded profiles are now available and undergoing further development to enable the range of profiles to be extended and production costs to be reduced. The market is already demanding the use of pultruded section as replacement for beams, roof supports and modular building components. With tightening fire and toxic fume emission requirements, the future for phenolics looks bright. Pultrusion Developments The major restrictions on pultruded components, which currently exist, may also be significantly reduced over the next few years. With very few exceptions, products must be parallel sided and have weaker transverse mechanical properties than those obtainable in the axial orientation, due to the inherent fibre alignment. Thermoplastics have already shown dramatic productivity increases but also offer the possibility of post forming sections into non-parallel sided shapes. Similarly, the introduction of the pullwinding variants provides the ability to accurately position transverse fibres thereby redressing the balance with the axial performance. Filament Winding High speed precise laying down of resin impregnated continuous fibres onto a mandrel is the basis of the filament winding process (figure 4). Pressure vessels, pipes and drive shafts have all been manufactured using filament winding. The mandrel can be any shape that does not have re-entrant curvature, although it is possible to remove the component from the mandrel before it has cured and use some other means of compaction to produce reverse curvature if required. Multi axis winding machines can also be used. The process is usually computer controlled and the reinforcement can be oriented to match the design loads. Components from small diameter tubes to 40 m, 13 tonne wind turbine blades have been manufactured using filament winding. One European company now manufactures commuter train carriages by a variation of the filament winding process. 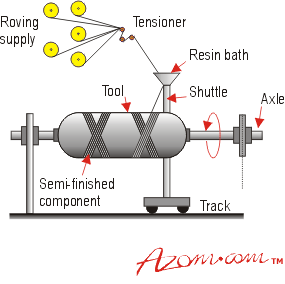 | Figure 4. Schematic of the filament winding process. | The fibres may be impregnated with resin before winding (wet winding), preimpregnated (dry winding) or postimpregnated. Wet winding has the advantages of using the lowest cost materials with long storage life and low viscosity. The prepreg systems produce parts with more consistent resin content and can often be wound faster. Materials Glass fibre is the fibre most frequently used for filament winding, while carbon and aramid fibres are also used. Most high strength critical aerospace structures are produced with epoxy resins, with either epoxy or cheaper polyester resins being specified for most other applications. The ability to use continuous reinforcement without any breaks or joins is a definite advantage for filament winding, as is the high fibre volume fraction that is obtainable (60-80%). Only the inner surface of a filament wound structure will be smooth unless a secondary operation is performed on the outer surface. The component is normally cured at high temperature before removing the mandrel. Finishing operations such as machining or grinding are not normally necessary. Prepreg Moulding Prepreg moulding is in many respects the next step up from hand lay up. With prepreg moulding the resin content of the finished component can be accurately controlled, which cannot always be said for hand lay up. Also, woven or unidirectional fibre reinforcements are used, rather than chopped strand mat. The reason is that they be aligned in the required orientation. However, prepreg materials are not cheap and they usually require oven curing and vacuum bag or autoclave moulding to take full advantage of their properties. The typical arrangement for vacuum bag moulding is shown schematically in figure 5. Prepreg moulding is still used extensively in the aerospace industry. It is also the method used for the manufacture of large one off composite structures such as racing yachts and F1 racing car monocoques. 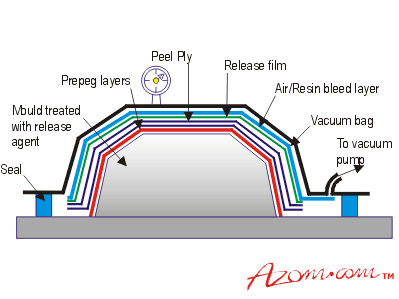 | Figure 5. Schematic of the vacuum bag process. | Materials The prepreg plies, containing the specified mix of resin, hardener, catalyst and reinforcing fibres, are cut to shape. The mould is treated with release agent and can be coated with a gel coat layer for the best surface finish. When the gel coat is tacky and partly cured, the prepreg plies are laid down in the appropriate orientation. With complex curves, the prepreg may not exhibit sufficient drape; then some modification of the ply shape is required. Once the plies are in position, for the production of a composite laminate the vacuum stack is then put in place. For the production of a sandwich structure, a film adhesive and the sandwich core material, which can be rigid foam or honeycomb, can be placed on top of the laminate and the laminate as well as a laminate core joint can be cured in one hit. Other Components The vacuum stack shown in figure 5 consists of a peel ply, release film, air/resin bleed layer, and the vacuum bag. The peel ply, frequently nylon, is removed last from the cured laminate and provides a clean, textured surface for bonding to. The air/resin bleed layer not only allows the vacuum to be maintained over the laminate, but also allows for some resin to bleed out of the laminate if necessary. Once the vacuum has been applied, the bagged component is either put in an oven or an autoclave. Autoclave moulding essentially involves vacuum bag moulding in a pressure cooker (with pressures up to 7 bar). As higher pressures are attained, thicker laminates and higher fibre volume fractions are possible. Processing Variables The temperature/time cycle used to cure the laminate is critical to achieve the optimum properties, as this will determine the resin flow and degree of cure. The process can be semi automated by using machines to cut the prepreg tape and robots to perform the lay up. Resin Transfer Moulding In the Resin Transfer Moulding (RTM) process, a low viscosity resin is transferred into a closed mould containing all the appropriate reinforcements and inserts as a preform. The air is normally evacuated from the mould, allowing the use of low resin injection pressures and epoxy moulds. Manhole covers, compressor casings, car doors and propeller blades have all been manufactured by RTM. Ford Motor Co recently demonstrated that the entire 90 piece front end of the Escort could be replaced by a 2 piece RTM structure. Production cycle times were estimated to be less than 10 minutes. The RTM structure was stiffer and stronger than the steel structure, as well as 1/3 lighter. Characteristics of RTM Processing Traditionally associated with low volume parts manufacture and low fibre contents, RTM research has undergone significant investment in the last few years. Cycle times of less than 3 minutes with fibre contents of over 50% by volume can already be demonstrated. For complex or large components, a cycle time of one to two hours is typical. Continued development is anticipated to result in a process capable of producing small components in less than one minute, with fibre contents approaching 60% by volume. RTM Developments Resin suppliers are formulating low viscosity systems dedicated to enabling faster, more controllable mould fill. Reinforcement suppliers are constructing fabrics with high fibre contents, formability and minimum resistance to resin flow. Automotive components, which are more structural in nature than body panels, such as chassis and subframe members, can be produced on modified Resin Injection Moulding (RIM) equipment using, RTM principles. The production volumes will be an additional bonus to complement existing advantages such as the potential for moulded in inserts, foam cores, good quality surface finish and tight tolerances. Moving the process into higher performance applications involves redesigning resins to have lower viscosities to compensate for diffusion through higher densities of fibre. The development of fabric forms compatible with higher process speeds and injection pressures will also hold the key to expansion and growth of RTM technology. The aerospace industry also has a keen interest in processing routes to compete with autoclaving, which is normally used for very high quality, low volume components. RTM may approach this requirement with considerable cost reductions, estimated at 40%. In response top this, autoclave technology is becoming more highly automated and less labour intensive, with the use of prepreg cutting, stacking and handling facilities. |