Introduction
Acrylated-epoxidized soybean oil (AESO) renders thermosetting compounds by thermal polymerization or copolymerization [1, 2]. These reinforced polymeric composites are useful in infrastructure, automotive, construction, aircraft, military, and electronic industries [3]. These materials have to be prepared first from low molecular liquid molding resins having functional groups for cross-linking. Subsequently, these resins are mixed with initiator (free radical or ionic), catalyst, reinforcing compounds as bamboo, henequen, hemp, cellulose, glass fiber, carbon or carbon nanotubes [4], and they are cross-linked to give a final cured composite [5]. We have studied electrical properties of composites prepared from thermal (free radical) cross linking of AESO and poly(AESO-co-butyl methacrylate) with carbon black (CB) [6]. Composites were obtained with a CB percolation concentration of 4% and 1.2% respectively. These composites had interesting electric properties; they showed a very low CB percolation concentration and an enhancement in conductivity in comparison with commercial polymers and copolymers as polyethylene, polystyrene [7] and polybutadiene [8]. However, they were insoluble, sticky and intractable materials. The main goal of this work was to study the gamma (γ) radiation effect on the cross-linking reaction of AESO for obtaining stable, homogeneous and definite shaped (the shape of the reaction tube) polymers and composites, as an alternative to thermal molding resins method, without using temperature, solvents, chemical initiators or pre-polymerization. It is well known that both cross linking and degradation process take place during polymer irradiation. These reactions are found to considerably affect the physical and mechanical properties [9] of a material. In this work, we also studied if both effects were present in the synthesized polymers, and we evaluate two tribological properties [9], friction and scratch resistance, which are sensitive to small changes in cross-linking density.
Experimental Procedure
Materials
Acrylated-epoxidized soybean oil (AESO) was purchased from Sigma-Aldrich Co. and it was used without further treatment. Toluene and acetone (Sigma-Aldrich Co.), used for washing the final products and to eliminate unreacted oil, and tetrahydrofuran (Sigma-Aldrich Co.) used for dispersing carbon black, were all analytical grade. Carbon black (CB) Vulcan XC72 (30 nm), was obtained from Cabot Co., USA.
Instruments
A 60Co γ-rays Traselektro LGI-01 working at a dose rate of 3.6 kGy/h was used for irradiation. AESO and their products, and the following of changes in structure were characterized using a Spectrophotometer FT-IR Nicolet Avatar 360. Samples were mixed with KBr and pressed at 8 tons for 2 min. in order to produce transparent plates. Solid (CPMAS) and liquid (using CDCl3 as the solvent) 13C NMR at room temperature, were obtained on a Bruker Avance 300 MHz spectrometer operating at a resonance frequency of 75 MHz. Differential scanning calorimetry and thermogravimetric analysis were performed on a TA Instruments SDT Q600, at a heating rate of 10°C/min, from 30 to 600°C and on a Perkin-Elmer DSC7 at the same heating rate, but from -30 to 300°C in nitrogen atmosphere. Surface morphology of polymers was examined with a scanning electron microscope (SEM, JSM-5900LV) without conductive covering on the samples. In order to evaluate the friction and scratch properties, all samples were cut to the same size (1.5 cm diam. x 0.5 cm thick) and polished manually on a flat surface using 600 grit silicon carbide paper until no defects on both surfaces were observed. For friction coefficients a friction attachment to a Q-TESTTM/5 mechanical testing machine, on a Teflon surface, at 150 mm/min testing speed and a nominal weight of 0.4309 Kg were used. Scratching evaluations were taken on a Swiss micro-scratch tester (MST) with the depth resolution of ± 7.5 nm. A diamond indenter with a point radius of 200 μm was applied in a progressively increasing (from 1 to 15 N forces) loading rate of 10 N/min and a scratch speed of 3.0 mm/min on a length of 10 mm.
Irradiation
1 g of AESO samples were weighed inside a 8 mL glass Pyrex, non-sealed, vial (2.0 cm diam. x 6 cm high) and irradiated at 12, 24, 74, 110 y 340 kGy in the presence of air, and without solvent or initiator. After irradiation, the complete solid products were shaken for 1 h with toluene and then with acetone. Products were decanted and dried under vacuum for 4 h. Only one composite was prepared in order to probe the viability of the irradiation method for producing them in a given shape. Complete characterization, including electrical properties measurements, will be discussed in a future paper together with the complete study of AESO-MAB copolymerization by gamma irradiation [6]. This polyAESO+CB(4% w) composite was prepared as follows: 1 g of AESO was weighed inside a 4 mL glass Pyrex vial, 2 mL of THF was added and they were shaken until complete dissolution. Then, 0.04 g of CB was added, dispersed using an ultrasonic processor UltrasonikTM 28X (50/60Hz) for 40 min. [6] and irradiated at 110 kGy. Composite was washed with toluene, then with acetone and finally dried under vacuum as described for irradiated polyAESO.
Results and Discussion
AESO started to polymerize at 12 kGy yielding an insoluble semisolid product (a semi polymerized compound) that continues polymerizing, until a solid product was obtained after 6 h of irradiation. Figure 1 shows the infrared spectra of polyAESO irradiated at 12 kGy. The important signals correspond to residual double bonds at 1640 cm-1 (C=C) and 991cm-1 (=CH2) that diminish in intensity as radiation dose increase. Double bonds signal (Figure 1) are still present at 24 kGy but the polymer is now solid, insoluble, transparent and cylinder shaped (Figure 2) with a rugged surface (Figure 3). Higher radiation doses (110 and 340 kGy) were used in order to evaluate the stability of the polymer. At 340 kGy it does not suffer degradation nor structural modification, but is a highly cross-linked polymer as the IR spectra shows, since double bond signals are gone (Figure 1). Polymers obtained by irradiation do not have any glass transition (Tg) in the temperature range from -30 to 300°C. Scala and Wool [1], reported that glass transition temperature of triglycerides with acrylate functionality (AESO, for example) increases approximately linearly (-50 to 92°C) with cross-linking density induced thermally. In this case, γ-radiation produces, since the beginning (12 kGy), a higher cross-linking effect on AESO.
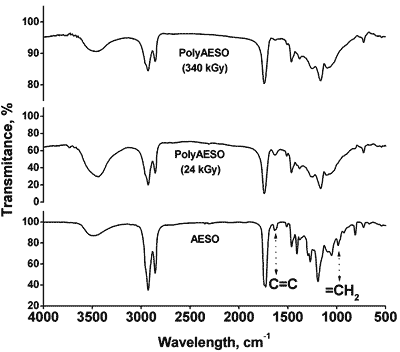
Figure 1. Disappearance of double bond signals as irradiation dose increases.
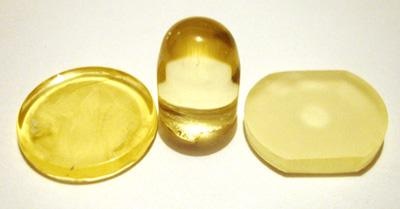
Figure 2. Image of unpolished polyAESO irradiated at 24 (left and middle) and polished 340 kGy (right).
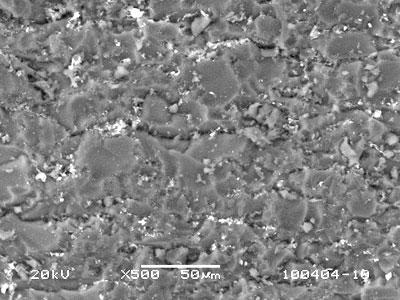
Figure 3. Micrograph of PolyAESO obtained at 24 kGy.
PolyAESO at any radiation dose (from 24 to 340 kGy) started to decompose in only one step, 15°C before (considering that the compounds have lost 10% of original weight) the AESO monomer does (Figure 4). This indicates that cross-linking diminished the free movement of segments of triglycerides chains, increasing the entropy of the system. This could also be the explanation for the difficulty on achieving a high thermal polymerization, which yields an intractable soft rubber [2]. No other decomposition temperature was detected by increasing the irradiation dose, meaning that no subproducts originated by chain scission reactions took place.
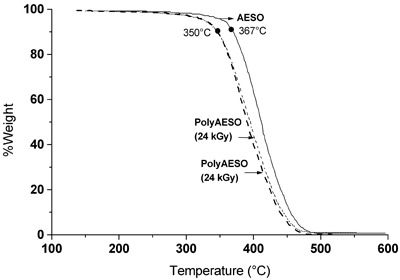
Figure 4. Cross linking reaction effect on thermal stability of polymeric products obtained at different irradiation doses, in comparison with the monomer AESO.
Liquid (CDCl3) 13C RMN spectra for AESO were taken in order to compare important structural changes depending on irradiation dose. Figure 5 shows the 13C NMR spectra of AESO and polyAESO irradiated at 340 kGy. Around 130 ppm are the signals corresponding to unsaturated (acrylic) carbons that reduce dramatically when it has been irradiated at 340 kGy, as we can appreciate in its solid 13C RMN spectrum (Figure 5). Additionally, the signals for polyAESO are wider, typical behavior of an important anisotropy of chemical shielding effect caused by a high cross-linking in polymers.
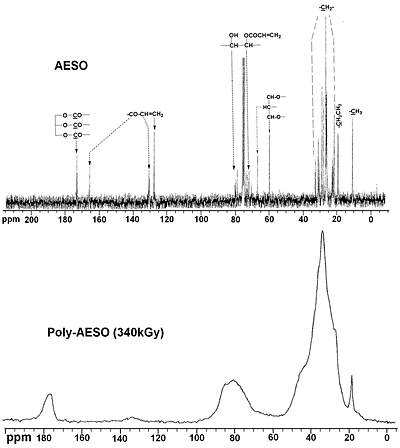
Figure 5. NMR spectrum showing the cross linking effect induced by γ-radiation (340 kGy) by disappearing signals corresponding to double acrylic bonds (around 130 ppm).
We evaluated two properties, scratching and friction, that allow to test the samples as they were obtained. These properties were very sensitive to small differences in cross-linking density [9]. The scratch test [10-12] consists of deforming the surface by indentation under load of a moving diamond tip. The applied load could be held constant, increased continuously or increased stepwise. Figure 6 shows curves of penetration depth (Pd) as a function of the applied force in the progressive mode for polyAESO prepared at different doses. Higher doses cause a decrease in the penetration depth, independently of the applied force. A hardening due to the cross-linking increase with the irradiation dose could produce this scratching resistance. PolyAESO´s obtained at 110 and 340 kGy show the same penetration depth, meaning that at 110 kGy maximal cross-linking has been reached. No evidences of degradation at 340 kGy were given by scratch evaluations. If some degradation were presented in PolyAESO, resistance to be penetrated would diminish (increase of penetration depth) [9].
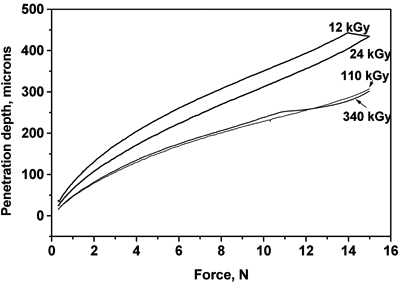
Figure 6. Penetration depth evaluations of PolyAESO irradiated at different doses as a function of the applied force.
Usually friction and scratch resistance are two tribological properties that are opposite to each other. A poor scratch resistance, as in Teflon, accompanies low friction values [11]. Hence, we could expect that the highest dose polyAESO will exhibit a lower friction. As can be seen in Figure 7, static friction decreases initially as irradiation dose increases, reaching almost a plateau at 24 kGy. Friction depends on alteration of material combinations and surface roughness. For fully packed and well ordered polymers, friction has been found to be lower than less ordered and amorphous material [13, 14]. A possible explanation for changes in friction values at different radiation doses is that γ-ray high energy is producing better packing polymer chains. Figure 8 shows the micrograph of polyAESO obtained at 340 kGy as an uneven surface with smaller domains than those in polyAESO irradiated at 24 kGy. This reduces the area of contact and the friction of the polymer produced at higher radiation dose [14].
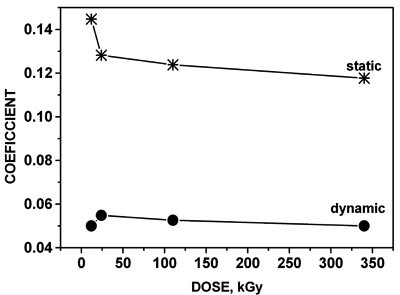
Figure 7. PolyAESO static and dynamic friction as a function of irradiation dose.
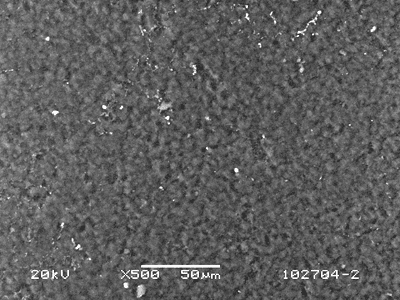
Figure 8. Micrograph of PolyAESO surface obtained at 340 kGy.
Finally, we prepared a polyAESO composite with 4% of CB by irradiation of the mixture previously described at 110 kGy. This composite was obtained with a vial shape; however, the presence of CB affects some properties of the compound. It looks more like a rubber than a rigid polymer. It is well known that additives can really affect mechanical properties, depending on the concentration, particle size, dispersion, and interaction with the matrix, among others. So, we need to consider the effect of gamma irradiation and cross-linking effects on the properties and stability of this kind of composites for future work.
Conclusions
AESO was irradiated from 12 to 340 kGy producing soft and very stable polymers; with increasing in cross-linking effect as γ-radiation dose was increased. No degradation was detected even at the highest dose (340 kGy) applied according to IR, NMR and TGA analysis. Two tribological properties were studied and they were susceptible to changes produced by irradiation doses. First, scratch penetration depth, in a progressive force experiment diminished as AESO was exposed to higher radiation dose. This is evidence of the hardening effect produced by a denser radiation-induced cross-linking. Scratch evaluation also shows that polyAESO obtained by γ-irradiation is a not a rubber as do the polyAESO thermally polymerized. Second, dynamic friction was not dramatically affected, but static friction was diminished in 19% after 110kGy. This effect of radiation-induced cross-linking on friction could be very important in composites prepared or cured at different doses. This γ-irradiation method allows to produce in situ, very stable AESO-based thermosetting polymers, copolymers and composites with definite shapes and properties that could be controlled by the polymer cross-linking as a function of irradiation doses.
Acknowledgements
Financial support of this work was provided by Universidad Autónoma del Estado de México (UAEM) (project No. 1806/2004A) and by Secretaría de Educación Pública (SEP) (project No. PROMEP/103.5/03/305) that made possible our stay (SHL and EVS) at LAPOM, University of North Texas. We would like to thank Prof. Witold Brostow and Dr. Dorota Pietkiewicz of LAPOM, for their valuable support with friction and scratching techniques.
References
1. J. La Scala and R. P. Wool, “Property Analysis of Triglyceride-based Thermosets”, Polymer, Vol. 46, (2005) 61-69.
2. J. Lu, S. Khot and R. P. Wool, “New Sheet Molding Compounds Resins from Soybean Oil. I. Synthesis and Characterization”, Polymer, Vol. 46, 71-80, 2005.
3. R. Wool, S. Kusefoglu, G. Palmese, S. Khot and R. Zhao, “High Modulus Polymers and Composites from Plant Oils”, US Patent No. 6, 121,398 (September 19, 2000).
4. M. In Het Panhuis, W. Thielemans, A. I. Minett, R. Leahy, B. Le Foulgoc, W. J. Blau and R. P. Wool, “A Composite from Soy Oil and Carbon Nanotubes”, International Journal of Nanoscience, Vol. 2, (2003) 185-194.
5. J. La Scala, J. M. Sands, J. A. Orlicki, E. J. Robinette and G. R. Palmese, “Fatty Acid-based Monomers as Styrene Replacements for Liquid Molding Resins”, Polymer, Vol. 45, 7729-7737, 2004.
6. J. Mercado-Posadas, S. Hernández-López and E. Vigueras Santiago, ”Epoxidized-Acrylated Soybean Oil Polymers-based Composites with Low Electrical Percolation Threshold”, Adv. in Tech. Mat. and Mat. Proc. J., Vol. 8 No.2, 214-219, 2006.
7. A. Marquez, J. Uribe and R. Cruz, “Conductivity Variation Induced by Solvent Swelling of an Elastomer-Carbon Black-Graphite Composite”, J. of Appl. Polym. Sci., Vol. 66, (1997) 2221-2232.
8. R. San Juan-Farfán, S. Hernández-López, G. Martínez-Barrera, M.A. Camacho-López and E. Vigueras-Santiago, “Electrical Characterization of Polystyrene-Carbon Black Composites”, Phys. stat. sol. (c), Vol.2, 3762-3765, 2005.
9. W. Brostow, M. Keselman, I. Mironi-Harpaz, M. Narkis and R. Peirce, “Effects of Carbon Black on Tribology of Blends of Poly(vinylidene fluoride) with Irradiated and Non-irradiated Ultrahigh Molecular weight Polyethylene”, Polymer, Vol. 46, 5058-5064, 2005.
10. CSM Instruments, “Advanced Mechanical Surface Testing”, Applications Bulletin, No. 18 (2002).
11. W. Brostow, G. Darmarla, J. Howe and D. Pietkiewicz, “Determination of Wear Surfaces by Scratch Testing”, e-Polymers, No. 025 (2004) 1-8.
12. M. Dolores Bermúdez, W. Brostow, Francisco J. Carreón-Vilches, J. J. Cervantes and D. Pietkiewicz, “Wear of Thermoplastics Determined by Multiple Scratching”, e-Polymers, No. 001 (2005) 1-9.
13. Z. Burton and B. Bhushan, “Hydrophobicity, Adhesión, and Friction Properties of Nanopatterned Polymers and Scale Dependence for Micro- and Nanoelectromechanical Systems”, Nano Letter, Vol. 5, 1607-1613, 2005.
14. P. M. McGuiggan, “Friction and Adhesion Measurements between a Fluorocarbon Surface and a Hydrocarbon Surface in Air”, The Journal of Adhesion, Vol. 80, (2004) 395-408.
Contact Details
|