Electrification of transportation and increased use of renewable energy are commonly thought to be the most efficient strategies for achieving considerable reductions in greenhouse gas (GHG) emissions. Both of these strategies necessitate the development of transformational energy storage technologies. Solid-state lithium batteries (LBs) are among the most attractive energy storage technologies, as stated in the Batteries Journal.
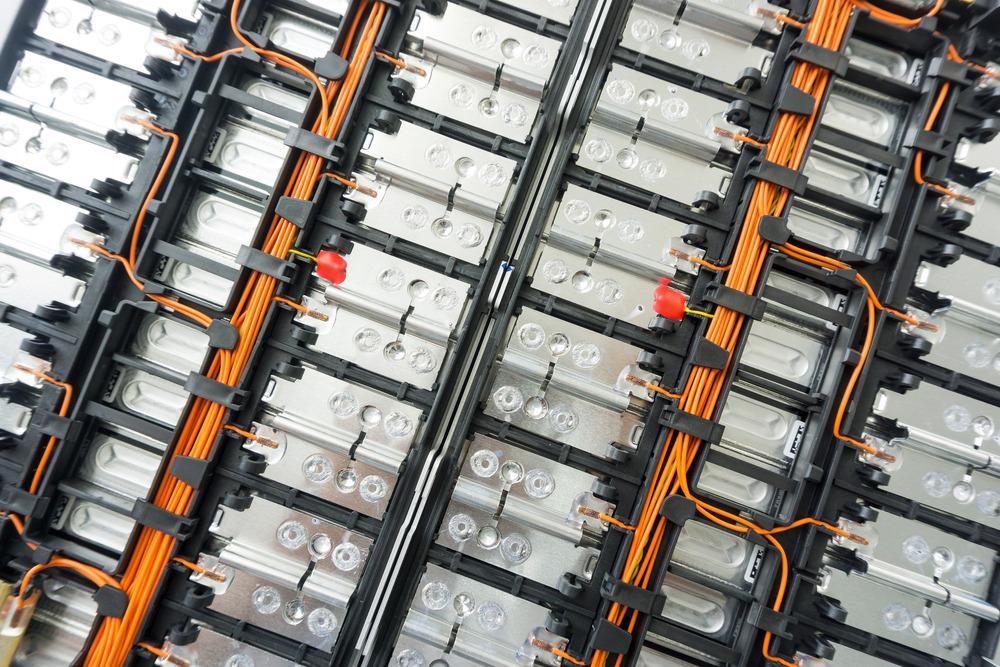
Yan, S., et al. (2021). Perovskite Solid-State Electrolytes for Lithium Metal Batteries. Image Credit: Smile Fight/Shutterstock.com
LBs are rechargeable batteries that were initially offered to the public in 1991 by Sony Corporation. LBs differ from traditional nickel-cadmium (Ni-Cd), lead-acid (Pb-acid), nickel-hydrogen (Ni-H2), and silver-zinc (Ag-Zn) batteries in that they have a substantially greater power density.
Renewable Energy using LBs
Organic liquid-state electrolytes, on the other hand, have high flammability and limited thermal stability, which is a typical safety problem for traditional LBs. Solid-state electrolytes (SSEs) can be used instead of liquid-state electrolytes to overcome this problem.
Energy or power cells with solid oxide electrodes and electrolytes may function over a wider temperature range and increase reactions at the cathode and anode, resulting in a faster discharging/charging rate. SSEs also offers a larger electrochemical window, making them more adaptable to high-voltage cathode materials and lithium-metal anodes, which can improve the energy density (up to 70%) and cycle performance of LBs.
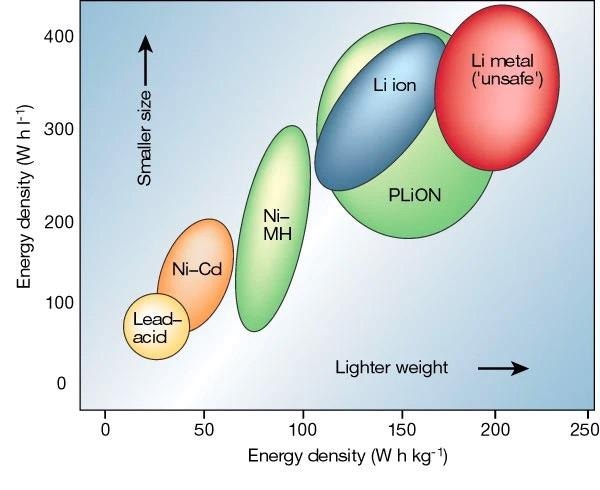
Ragone plot of lithium batteries. Image Credit: Yan, S., et al, Batteries Journal
SSEs can provide the safest battery with the highest power density to fulfill the need for electricity for air and surface vehicles when paired with a lithium metal anode and Ni-rich oxide ceramic cathode. Polymer, inorganic (e.g., ceramic-based oxide electrolytes), and hybrid electrolytes are all examples of SSEs. As an in-situ technique, free-standing polymer electrolytes might be created using appropriate crosslinkers and photopolymerization.
Fabricated gel polymer electrolytes have strong electrochemical characteristics and can make good interfacial contact with electrodes. Recent progress has been made in the field of polymer-based electrolytes. Unfortunately, their ionic conductivity is still restricted at ambient temperature.
Perovskites, NASICON-type, garnets, and LISCON-type inorganic oxide SSEs are the most common. Hybrid polymer-ceramic systems, like perovskites or LLTO, include ceramic fillers with garnets as a dispersion into the polymer matrix.
High Grain Resistance of LLTO
Despite its many appealing features, LLTO's high grain boundary resistance, which contributes to its low total ionic conductivity at ambient temperature, makes combining LLTO SSEs into LBs a serious difficulty. Both grains and grain borders impact overall ionic conductivity. The most common method used by researchers to enhance overall ionic conductivity for certain electrolytes is to change the composition of grain boundaries.
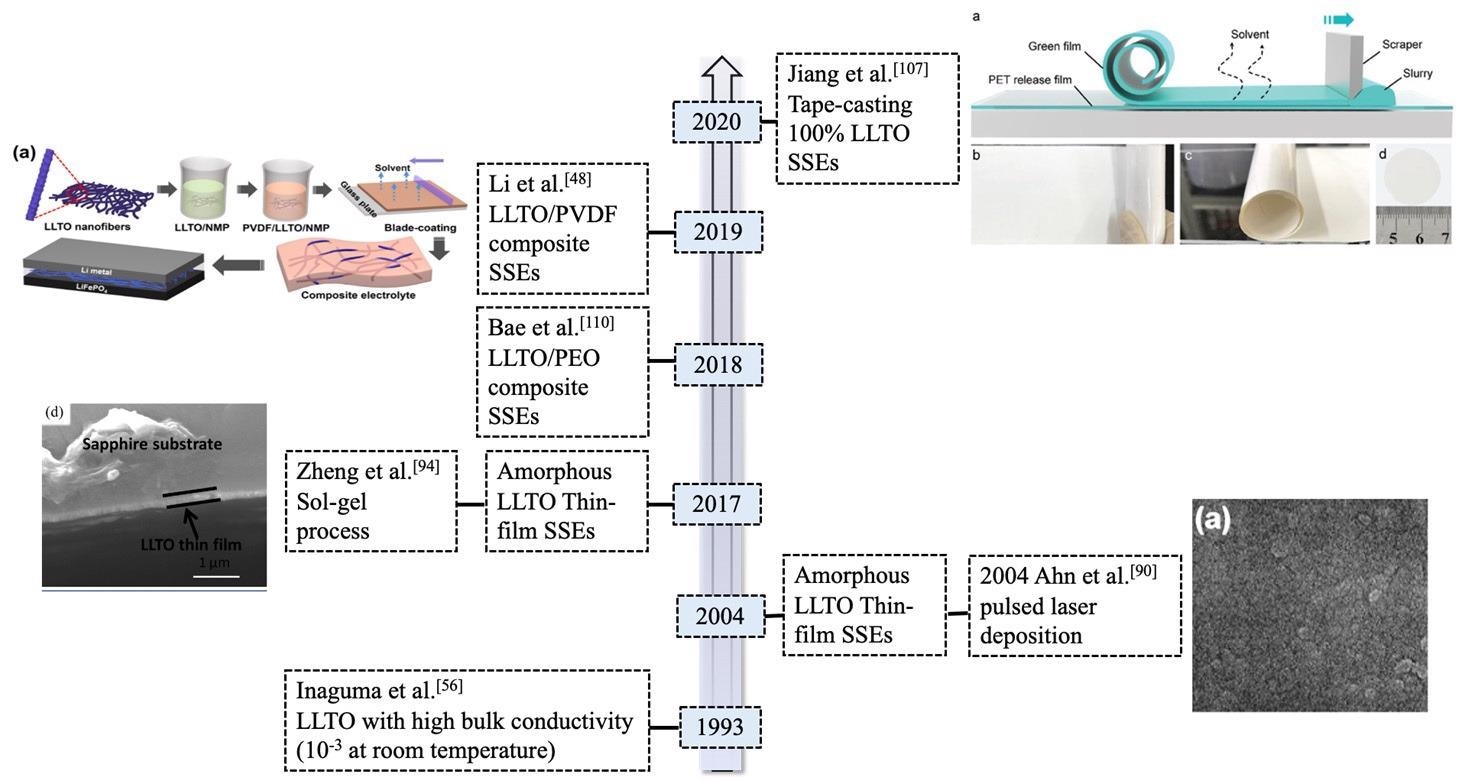
Timeline for the development of typical LLTO (La2/3-xLi3xTiO3) solid-state electrolytes (SSEs) in lithium metal batteries. Image Credit: Yan, S., et al, Batteries Journal
Another option is to increase the density of LLTO and minimize the grain size, as described in the following paragraphs. The change of LLTO's morphology such as random or arranged one-dimensional LLTO nanofiber is an effective technique to increase its overall ionic conductivity.
One-dimensional LLTO nanofibers featuring high surface-to-volume proportions can increase the quantity of continuous ion-conducting channels compared to LLTO particles.
Grain Boundary in LLTO
Grain boundary resistance takes place on the surface between crystallites in LLTO matrices, and it can obstruct the pathway of lithium-ion migration, lowering intergranular conductivity. The mismatch between LLTO grain boundaries, which are made up of a Ti-O binary combination with a 2–3 unit-cell thickness, is referred to as lattice mismatch.
When most grain borders between LLTO matrices have structural and chemical variations to compensate for random orientation of nearby LLTO grains, lattice mismatch occurs [73]. Because it is not energetically advantageous for lithium-ion accommodation or migration, grain boundary conductivity is weak.
Adjusting the LLTO grain boundary compositions, as previously noted, might be an efficient strategy to lower grain boundary resistance. The introduction of dopant components into the A-sites and/or B-sites in the crystalline form of LLTO via the creation of more intrinsic defects is one successful method. An amorphous coating or valence ions is another option.
Layer Coating
Most studies use a metal-layer coating (e.g., Au, Ag, and Cu) as an intermediate film in symmetric cells to safeguard LLTO from responding with a lithium-metal anode for conductivity measurements. One strategy that has proven successful using garnet-type electrolytes is to utilize an interfacial layer with increased stability (kinetic or via the development of stable SEI such as PEO-based electrolyte films). The use of metal oxides is one option.
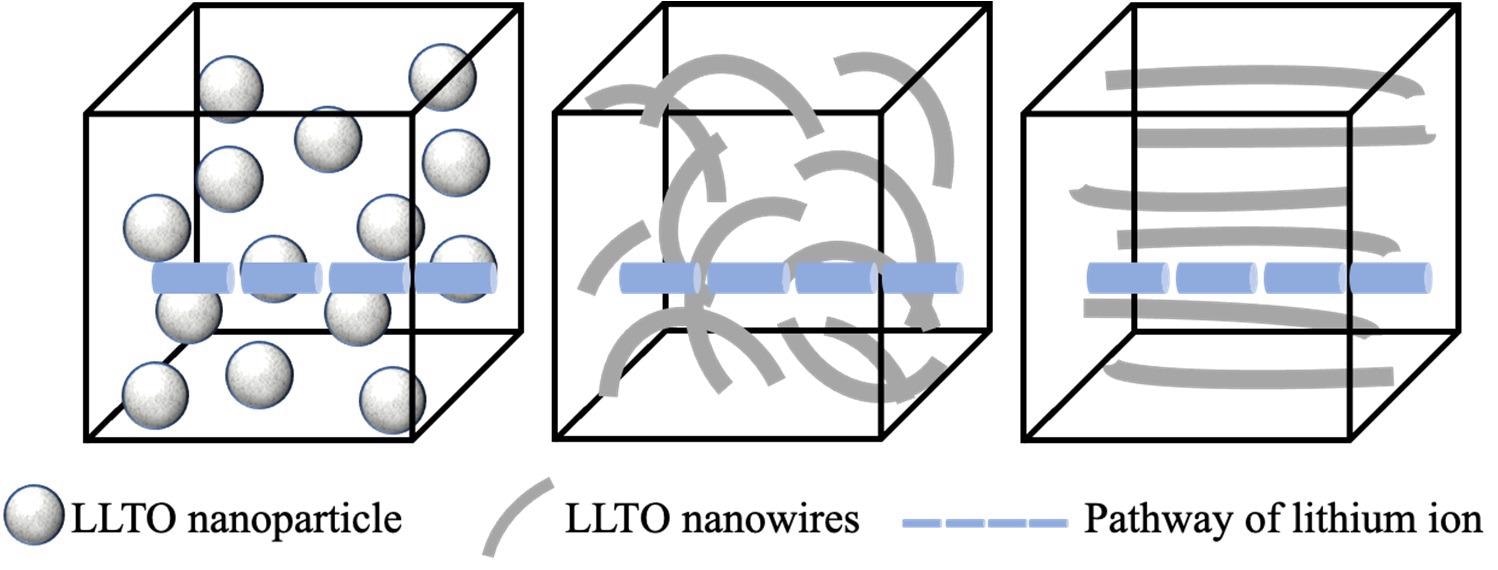
Schematic images of pathways of lithium ions when LLTO as (a) isolated nanoparticles; (b) random nanowires; and (c) well-aligned nanowires. Image Credit: Yan, S., et al, Batteries Journal
There are few reports of LLTO electrolytes being made with the layer casting technique. Some have published on how to change several factors such as dispersant percentage, binder to plasticizer ratio, actual sum of binder and plasticizer, and dry conditions to improve slurry composition.
In terms of mechanical integrity and stacking stress, LLTO ceramic thin films are fragile, making battery construction difficult. A stacking pressure that maintains excellent connections between layers while causing minimal damage to SSEs must be applied. SSEs may be protected from cracking and fracturing by buffer layers such as nickel-coated sponges.
References
Yan, S., et al. (2021). Perovskite Solid-State Electrolytes for Lithium Metal Batteries. Batteries Journal. 7 November 2021. https://www.mdpi.com/2313-0105/7/4/75
Disclaimer: The views expressed here are those of the author expressed in their private capacity and do not necessarily represent the views of AZoM.com Limited T/A AZoNetwork the owner and operator of this website. This disclaimer forms part of the Terms and conditions of use of this website.