A paper recently published in the journal Energies demonstrated the effect of potassium salts on the pyrolysis behavior of different biomass.
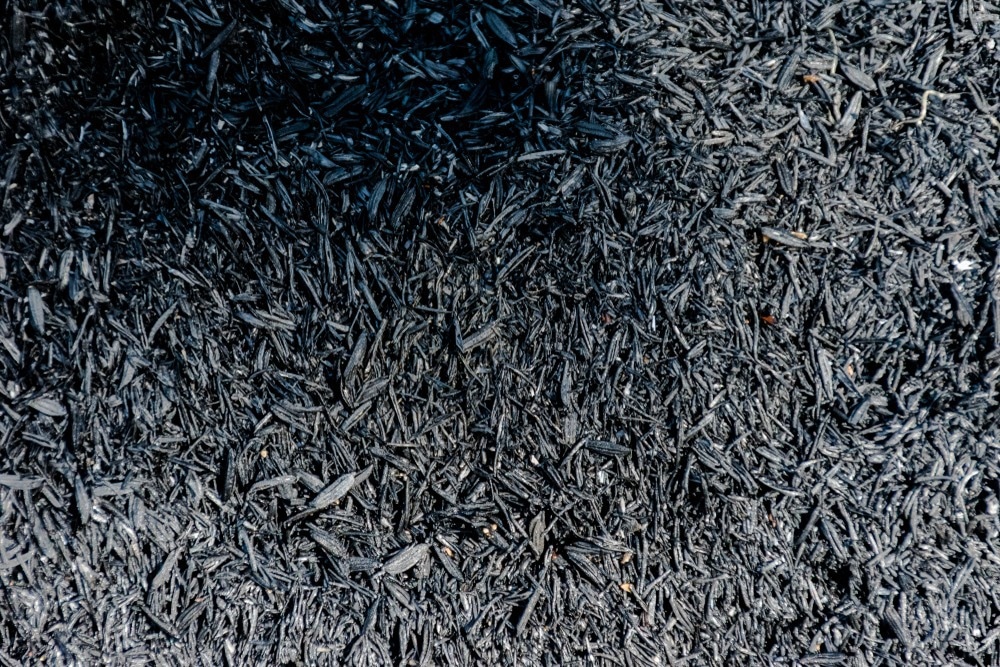
Study: Effect of Potassium Salts on Biochar Pyrolysis. Image Credit: StorKiss/Shutterstock.com
Background
The growing dependence on fossil fuels for energy generation has adversely affected the environment and human health in many ways, such as by increasing the emission of greenhouse gases. Biomass is often considered a suitable alternative to fossil fuels for energy generation. In the United States (US), the use of wheat straw and pine as biomass feedstocks is more suitable since they are native and abundant in the country.
Alkali pretreatment is one of the chemical pretreatment technologies used for different types of lignocellulosic biomass to improve pyrolysis efficiency. The chemical and physical mechanisms of the transformation and release of inorganic elements during biomass conversion are complex.
For instance, the release of potassium depends on the form of potassium and the other ash-forming elements present in the fuel/biomass and operational conditions. Similarly, high chlorine contents facilitate the release of potassium during char combustion, whereas high silicon contents capture the potassium efficiently in the silicate structures, specifically at the advanced stages of char oxidation.
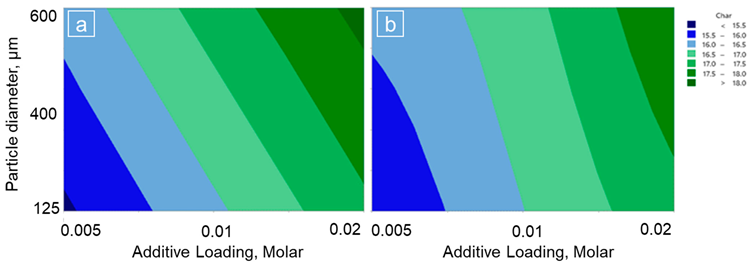
The contour plots of wt. % char remaining with the additive loading (molar) and particle size (μm) for; (a) pine wood with KHCO3; (b) pine wood with KNO3. Image Credit: Wongmat, Y et al., Energies
The Study
In this study, researchers investigated the influence of potassium-based additives on various biomass feedstocks by evaluating the pyrolysis efficacy in terms of conversion and activation energy (AE).
Potassium nitrate (KNO3) and potassium bicarbonate (KHCO3) were utilized as additives in the study. Researchers also investigated the influence of different operating parameters, such as additive types and loadings, heating rates, and particle sizes, on char production.
Wheat straw and pine wood were used as the starting materials, which were ground and sieved to obtain wheat straw particles with sizes ranging from 125–150 µm to 600–630 µm and pine wood particles with sizes 400-425 µm to 600–630 µm. Subsequently, the fuels were dried in an oven for 24 h at 105 oC to decrease the moisture content.
KHCO3 and KNO3 were dissolved mechanically in distilled water to prepare their respective liquid solutions with 0.005 M, 0.01 M, and 0.02 M concentrations. The biomass was synthesized by treating the five g of the prepared feedstocks with the potassium solutions and then sonicating the treated samples for 40 min. Subsequently, the as-prepared samples were filtered and heated for 24 h at 105 oC before pyrolysis.
During pyrolysis, the KNO3 and KHCO3-treated samples were heated from room temperature to 700 oC, and nitrogen with a 100 mL/min flow rate was utilized as the carrier gas. An untreated feedstock sample was used as a control, and its results were compared with the results of the alkaline-treated samples to ensure the reliability of the study and determine the effects of operating parameters.
A thermogravimetric analyzer (TGA) was used for the thermal characterization of the samples. The design of experiments (DOE) approach was implemented using the Minitab software to investigate the effect of the operating parameters on the char yields. Moreover, a model-free analysis method, Flynn–Wall–Ozawa (FWO) model, was utilized to measure the AE values of the potassium-treated and untreated feedstocks.
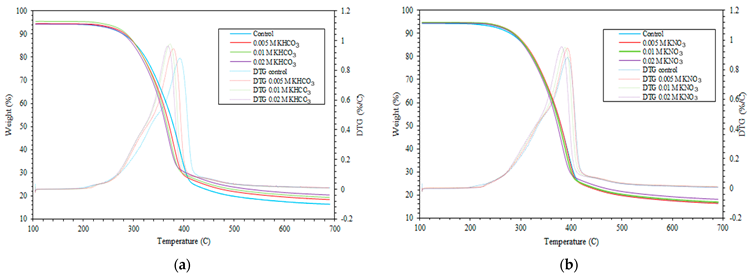
TGA profile of: (a) large pine wood impregnated with KHCO3; (b) large pine wood impregnated with KNO3. Image Credit: Wongmat, Y et al., Energies
Observations
Char obtained from the KNO3 and KHCO3-treated pinewood samples increased in correspondence with the increasing additive loading and particle size. The decomposition of the potassium-treated and untreated biomass during the non-isothermal pyrolysis can be divided into three stages.
Loss of moisture and some volatile components were observed in the first stage, with the reaction temperature ranging from room temperature to 150 oC. In the second stage, with a 150-400 oC reaction temperature, the primary constituents of the biomass, such as hemicellulose and cellulose, were decomposed. The lignin was decomposed slowly in the final stage of pyrolysis through thermal degradation at 160–900 oC.
Biomass/pine wood decomposition was kinetically faster at the second stage in samples with higher additive concentration compared to samples with lower additive concentration or untreated samples, leading to a higher conversion of high potassium-containing samples to gas and a reduction in the biochar yield.
However, the decomposition of pine wood with lower potassium concentration was kinetically faster in the final stage of pyrolysis, leading to a higher char production. Alkali impregnation improved the reactivity of both cellulose and hemicellulose due to the catalytic effect of potassium.
An increase in additive loading and reduction in particle size for wheat straw resulted in increased biochar production. However, the biochar production decreased when the particle size increased from 125 µm to 600 µm due to the heat transfer limitation that occurred during the pyrolysis of wheat straw.
During the second stage of pyrolysis, the reaction of wheat straw containing higher additive concentration was kinetically slower compared to samples with lower additive concentration, indicating that the char yield from both KNO3 and KHCO3-treated samples increased with the rising additive concentration.
However, the reaction of the treated wheat straw samples was faster compared to untreated samples when the reaction temperature was increased above 400 °C, leading to a higher weight loss at the final temperature. The biomass structure changed from amorphous to crystalline due to the addition of potassium-based additives in the wheat straw, which shifted the temperature range of devolatilization rates of biomass to higher values.
The AE values obtained for non-isothermal pyrolysis of KHCO3-treated large pine demonstrated a small variation with conversion. Additionally, the AE values obtained from the untreated sample were significantly higher compared to the other KHCO3-treated samples. The lowest AE values were observed in samples with 0.01 M KHCO3.
The AE values varied in the three biomass degradation stages, with the values decreasing in the first stage, remaining constant in the second stage, and increasing significantly in the final stage due to the improvement in the aromatic carbon structure in the biomass.
Similarly, the AE values for KNO3-treated large pine wood also displayed a similar trend as KHCO3-treated samples, with higher values observed in untreated samples compared to potassium-treated samples and the lowest values observed in samples containing 0.02 M KNO3.
In the first and second biomass decomposition stages, the AE values of the KNO3-treated large pine reduced slightly and fluctuated, while in the final stage, the values increased sharply. The sharp rise in AE values was attributed to the rise in crystallinity and highly cross-linked biomass, complex structures of lignin-derived char at higher temperatures, and unburnt solid residual, tar, and other carbonaceous matter.
The AE values for non-isothermal pyrolysis of wheat straw treated with different concentrations of KHCO3 were measured. A reduction in AE values was observed in heat straw with higher potassium content compared to untreated wheat straw. The lowest activation values were observed in samples containing 0.01 M KHCO3.
In the second and first stages of decomposition, the AE values were constant, and the variations between the samples were extremely large. Meanwhile, in the final stage, the AE values increased significantly due to the lignin-derived aromatic compound decomposition and low reactivity of wheat straw pyrolysis at higher temperatures.
AE values for KNO3 treated-large wheat showed a similar trend as KHCO3-treated wheat straw. Moreover, the AE values of wheat straw with different KNO3 concentrations were almost similar in the first and second stages of pyrolysis. In the last stage, the values increased significantly at higher temperatures.
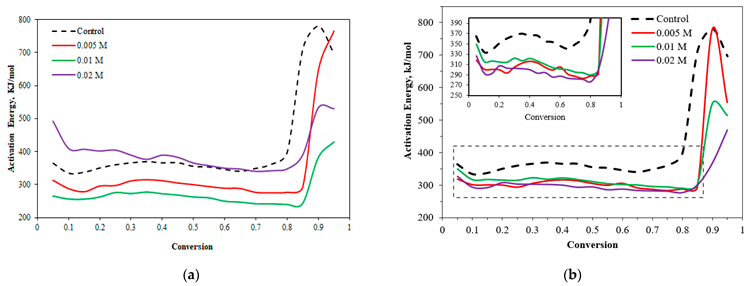
Activation energy profile for non-isothermal pyrolysis of: (a) large wheat straw impregnated with KHCO3; (b) large wheat straw impregnated with KNO3. Image Credit: Wongmat, Y et al., Energies
Conclusion
Although the char yield in both biomass materials increased with the increasing concentration of potassium-based additives, the char yield of the treated wheat straw was lower compared to its untreated form.
The AE values were reduced in all potassium-treated samples compared to their raw/untreated forms, excluding the 0.02 M HCO3-treated wheat straw samples. AE values of the treated wheat straw ranged from 277 to 402 kJ/mol, and treated pine wood ranged from 250 to 308 kJ/mol.
To summarize, the findings of this study demonstrated that the addition of potassium salts to biomass can effectively promote the catalytic effect of pyrolysis.
Source
Wongmat, Y., Wagner, D.R. Effect of Potassium Salts on Biochar Pyrolysis. Energies 2022.https://doi.org/10.3390/en15165779
Disclaimer: The views expressed here are those of the author expressed in their private capacity and do not necessarily represent the views of AZoM.com Limited T/A AZoNetwork the owner and operator of this website. This disclaimer forms part of the Terms and conditions of use of this website.