In a paper recently published in the journal ACS Applied Engineering Materials, researchers introduced a scalable and simple technique for converting polypropylene (PP) surgical mask wastes into carbon fibers doped with sulfur that can show high sorption capacity for carbon dioxide (CO2) and selectivity against N2.
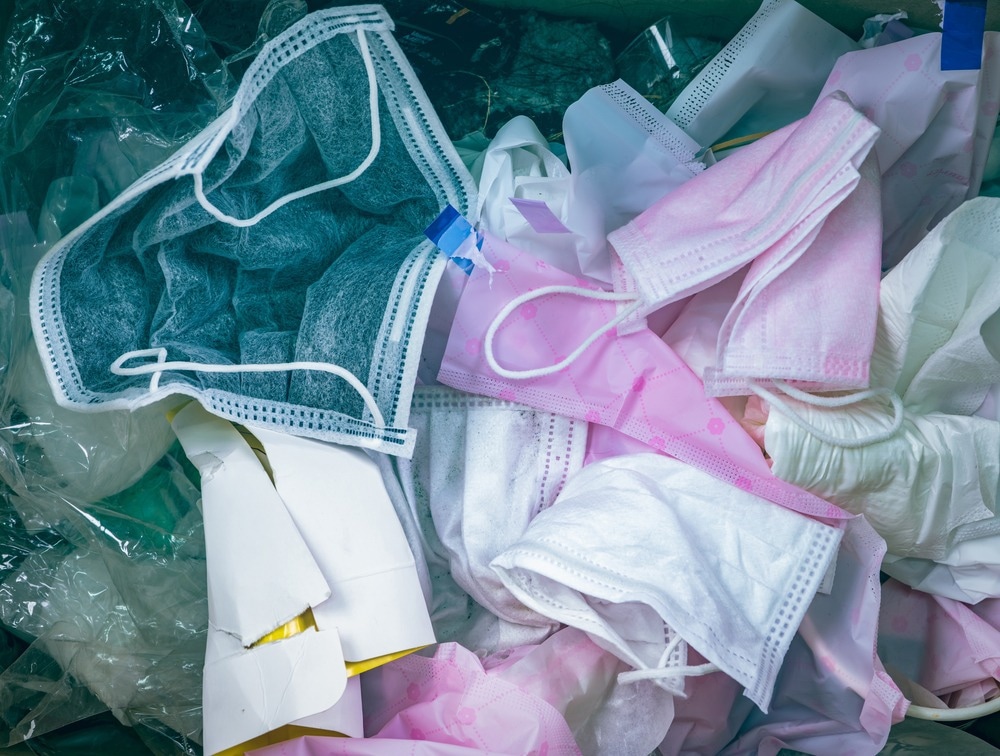
Study: Upcycling Mask Waste to Carbon Capture Sorbents: A Combined Experimental and Computational Study. Image Credit: Fahroni/Shutterstock.com
Background
Massive greenhouse gas emissions from various industries, such as manufacturing, transportation, and electricity generation have had detrimental effects on the environment, including faster global warming, extreme weather, and respiratory diseases from air pollution. Furthermore, the increasing CO2 concentration would lead to global health and environmental crises.
Therefore, the continuous efforts in developing CO2-capturing technologies have led to the commercial-scale implementation of liquid sorbent systems in manufacturing plants. However, these are often accompanied by corrosion, release of harmful byproducts, and heavy sorbent regeneration penalties.
Recent studies to reduce CO2 emission have included the use of metal organic frameworks (MOFs), biomass precursor carbonization, as well as sulfur-doped (S-doped) carbons. Another study exhibited the production of hollow carbon fibers using PP fibers followed by microspore formation through an activation step. Such work can resolve the concerns due to enormous quantities of mask waste generated during the COVID-19 pandemic, resulting in significantly increased manufacture and consumption of single-use plastics derived from PP.
About the Study
In the present work, the team employed sulfonation chemistries to cross-link the PP fibers derived from PP masks and converted them into S-doped carbon fibers through the addition of sulfonic acid to the backbone of the polymer.
This study followed cutting the surgical masks to retrieve the outer nonwoven layers to which 98% sulfuric acid was added. An electric muffle furnace was then used to heat these materials at 145°C. This was then followed by washing the samples with deionized (DI) water, drying, and carbonization in a tube furnace to 800°C in an N2 atmosphere.
Using a PerkinElmer Frontier attenuated total reflection Fourier transform infrared (FTIR) spectrometer, the chemical contents of mask samples were identified. Furthermore, the microstructures of masks following various processing phases, including carbonization and cross-linking, were examined using a scanning electron microscope (SEM). The carbonized masks’ elemental composition was determined by energy-dispersive X-ray spectroscopy (EDX). In order to determine the CO2 binding energy with relevant fiber structural motifs, density functional theory (DFT) simulations were used.
Observations
The FTIR spectra showed that the polymer backbone’s C-H stretch at a 2,920 cm-1 peak diminished with progressing sulfonation reaction. However, the FTIR spectra were observed to be nearly consistent after four hours, indicating the completion of the reaction. Moreover, the carbon yield plateaus reached around 40% after four hours of sulfonation, indicating that cross-linking at 145 °C using concentrated sulfuric acid was adequate to completely cross-link the PP fibers in the mask samples.
After cross-linking and carbonization processes, fibril structures can remain intact. However, PP-derived carbons can develop microporosity. Micropores were observed at longer sulfonation durations due to the increased amounts of cross-linking. Furthermore, increasing the sulfonation durations increased the heteroatom concentrations for sulfur and oxygen. Thus, the use of these carbon fibers as CO2 capture sorbents is enabled by the porous character and the heteroatom content, with the highest capacity of specific sorption totaling 3.11 mmol/g at 1 bar. Additionally, the materials also showed about 53 times higher selectivity towards CO2 compared to N2.
However, cross-linking for 12 hours showed decreased sorption capacity with marginally higher contained sulfur. These results suggest that the determination of CO2 capture efficiency also depends on the sulfur atoms’ local chemical environment inside the carbon structure. In order to understand their long-term usage and performance, the cycle stability of carbon fibers derived from PP masks for the desorption and adsorption of CO2 molecules was examined. It was observed that sorbent heating at 150 °C can entirely expel captured CO2 gas molecules, allowing the thorough regeneration of the expended sorbents. Additionally, the sorbents also exhibited high cycle stability, retaining more than 92% of their performance after over 35 cycles.
Conclusions
To summarize, the researchers produced carbon fibers using PP-based surgical masks by polymer precursor cross-linking at elevated temperatures in the presence of sulfuric acid. It was determined that sulfur atoms could be incorporated into the carbon matrix through a sulfonation reaction. Furthermore, by adjusting the sulfonation period, the sulfur doping amount in the carbon framework can be controlled.
Moreover, the CO2 sorption capacity can be enhanced by the sulfur heteroatoms, along with carbon-fiber selectivity, because of their advantageous polar interactions. Overall, the authors suggest that the production of CO2 sorbents from recycled mask wastes into S-doped carbon fibers is a scalable and straightforward alternative to address the environmental issues imposed by the increasing plastic pollution as well as atmospheric greenhouse gases.
Disclaimer: The views expressed here are those of the author expressed in their private capacity and do not necessarily represent the views of AZoM.com Limited T/A AZoNetwork the owner and operator of this website. This disclaimer forms part of the Terms and conditions of use of this website.
Source:
2022. Mark Robertson, Alejandro Guillen Obando, Bianca Nunez, Haoyuan Chen, and Zhe Qiang. Upcycling Mask Waste to Carbon Capture Sorbents: A Combined Experimental and Computational Study. ACS Appl. Eng.Mater. https://pubs.acs.org/doi/10.1021/acsaenm.2c00030