Only by careful measurement of their properties can materials be effectively and safely used. Until recently, it was only possible to assess fire behaviour by standard tests which provided but a simple grading of the materials tested. The data obtained from these assessments apply to the behaviour of the material inside the test apparatus and it is rarely possible to use such data to calculate the performance of the material in its end use environment. Such measurements are consequently referred to as ‘apparatus bound’. This situation has been greatly improved in recent years by the development of a range of measuring techniques for fundamental fire properties such as ignitability, flame spread, heat release, smoke production and toxic gas generation. All of the new tests provide data in engineering units that can be used in calculations and mathematical models which allow the designer, regulator or specifier to select and use materials safely in any end use environment. To appreciate the implications of these developments, it is necessary to understand what characteristics that influence the fire behaviour of materials and the mechanisms by which fire grows, and the threat to life it poses. Thermal Inertia Although the first property which usually comes to mind when considering fire is calorific value, the one which most influences initial fire behaviour is usually thermal inertia. This property is closely associated with another important property, comfort. Materials which are warm to the touch are widely used in buildings and vehicles where contact with the human body occurs. If a material is warm to the touch it is because the heat from our skin is transferred to the material and quickly raises its surface temperature. This occurs with materials which have low density, low heat capacity and low thermal conductivity. Conversely, materials with high density, high heat capacity and high thermal conductivity tend to feel cold to the touch because heat from the skin is quickly conducted into the body of the material and only has a small effect on surface temperature. Ignition A more dramatic effect is observed if human skin heat is replaced by an ignition source such as a match flame. It is difficult to heat the surface of the ‘cold to touch’ materials to their ignition temperature, whereas the ‘warm to touch’ materials are quickly raised to their ignition temperature, and once ignited, fire growth is rapid. Unfortunately, low thermal inertia materials are also highly desirable for other valuable applications such as heat insulation. The use of such materials is closely associated with human comfort requirements inside buildings and vehicles and, if not properly controlled, could represent a serious hazard to life in fire. Behaviour of Polymers in Fire Some synthetic polymers are popular with manufacturers because they can often be formed into complex end use shapes using simple moulding techniques. This valuable facility depends on the materials being thermoplastic. However, what can be formed by heat can also be deformed by heat and this can lead to initially solid materials moving their position in a fire. Deformation and melting can lead to increased fire spread. In particular, burning droplets can spread fire downwards and substantial acceleration of fire growth is often produced by the formation of pools of burning liquid beneath the item involved. This is a particular problem with domestic upholstery where thermoplastic deformation of polyolefin fabrics and the decomposition of polyurethane foams produce flammable liquids which significantly accelerate the fire growth rate. Growth of Fires Most people are familiar with the growth of fire in the open through experience with garden bonfires, but their first experience of fire inside a building would probably be in a life threatening situation. The difference in growth mechanisms between these two types of fire is so great that people's previous experience does not prepare them for what happens when they encounter a fire inside a building. The garden bonfire grows progressively as the flames from one item of fuel ignite the next. The heat and smoke rise harmlessly into the atmosphere. Inside a building the growth of fire follows a quite different process and is potentially much more dangerous. A wider understanding of the simple processes which control the growth of an indoor fire could greatly improve chances of safe escape. Flashover The fire inside a building will initially grow from the first item ignited in a similar way to the garden fire. However, the hot smoke produced will be trapped under the ceiling of the room and form a smoke layer. As the fire progresses, this smoke layer becomes deeper and hotter as more heat is ‘pumped’ into it from the burning products below. As the depth and temperature of this layer increase, some of its heat is released as thermal radiation downwards on to the products (and people) below. If the temperature of the smoke layer rises sufficiently, the intensity of the emitted radiation will become so high that other materials or objects in the room are heated to their ignition temperature and ignite virtually simultaneously. This phenomenon is referred to as flashover and produces a massive stepwise change in the size and hazard of the fire, fig 1. 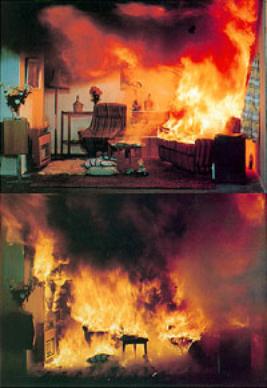 | Figure 1. Flashover in a domestic room (shown before and after) occurs at a heat release rate of about 1,000,000 Watts. | Measuring Heat Release Rates An important parameter in the induction of flashover is the rate of heat release from the initial burning item. In a small (domestic) room the heat release rate required for flashover is about one million watts. This is a very high level but it has been found that many domestic armchairs will quickly produce rates of heat release in excess of this level. The measurements of this parameter can be achieved using oxygen consumption calorimetry. In this method all combustion products from the burning items are collected by a canopy and passed into a duct in which the volume flow and oxygen concentration are accurately measured. In this way the amount of oxygen consumed can be measured on a second by second basis. For most materials the heat released for each kilogram of oxygen consumed is within ± 10% of 13 MJ. Using this, the heat release rate can be readily calculated from the rate at which the oxygen has been consumed. The typical full scale fire calorimeter at The Fire Research Station, Borehamwood (fig 2) has a tall chimney which provides extraction of the smoke by natural buoyancy. Most modern calorimeters, however, employ a horizontal duct and a powerful fan capable of extracting about 5 m3.s-1. 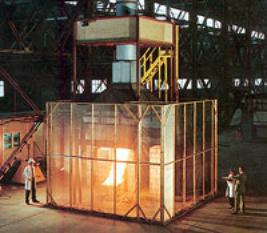 | Figure 2. A full fire scale calorimeter. | Whilst large scale calorimeters determine the heat release from a product or a system, small calorimeters exist with which the rate of heat release of materials can be measured on a unit area basis. The cone calorimeter uses a 100 by 100 mm sample to determine the heat release of materials, but in order to induce the release of heat, the material needs a certain level of heat input. This is achieved using a electrically powered cone-shaped heater above the specimen. Fire Behaviour of Materials The difference between the applied heat input and the induced heat output is critical in determining the fire behaviour of the material. Many materials ignite at a heat input rate of around 15 to 30 kW.m-2 but, in developed fires, levels of 50 kW.m-2 are common. In severe cases such as aircraft fires, levels of 100 kW.m-2 may be found. By comparison, the levels of heat released by materials can be 10 or even 20 times higher than the applied incident heat levels. It is this magnification of heat which induces growth in the fire. It can be seen therefore that the measurement of heat release rate is an important feature when characterising the expected behaviour of materials in fire. Smoke Production Since both small and large scale calorimeters involve the collection of all of the combustion products, it is a relatively simple matter to extend measurements of heat release to include the quantitative measurement of visible smoke and a range of selected toxic gases. Smoke is continuously monitored by an extinction beam photometer (light beam and photocell). The optical density so determined can be integrated with the volume flow in the duct to produce a quantitative measure of smoke production rate and total smoke yield. Smoke production can vary enormously from material to material. For example, burning polystyrene produces approximately 20 times more smoke than an equal mass of wood. Carbon Monoxide and Other Gas Emissions The principal toxic gas produced by fires is carbon monoxide and this gas can be monitored continuously by means of a nondispersive infrared gas analyser and, as with visible smoke, can be integrated with the volume flow in the duct to provide both production rate and total yield values. Other gases such as oxides of nitrogen, hydrogen chloride and hydrogen cyanide can all be found in fire gases depending on the materials involved and they can all be measured quantitatively using appropriate gas analysers attached to the calorimeters or by subsequent analysis from ‘grab’ samples taken periodically throughout the fire. In addition to these common fire gases, the full spectrum of chemical species present can be monitored by extracting gas samples for subsequent examination using gas chromatography and mass spectrometry. Fire Inhibitors Smoke and toxic gases released in fires are frequently the first things that victims encounter and are often responsible for delaying escape and early incapacitation. Such measurements are therefore of great importance. However, it must always be borne in mind that when smoke is released in a fire it does not induce the release of further smoke, and the release of toxic gas does not induce the release of further toxic gas. But when heat is released it can induce the release of more heat, more smoke and more toxic gas. Clearly, noncombustible materials such as mineral fibre can initially exhibit low thermal inertia without the attendant fire hazard, but combustible materials can also be made to behave more safely in fire by introducing agents which interfere with the combustion process. Types of Fire Retardant Materials Some additives release gaseous species when heated which pass into the flame zone and interfere with the flame chemistry tending to extinguish the flames; these are referred to as vapour phase fire retardants. Some additives achieve protection in the solid phase by inducing the formation of a protective coating inert layer of solid material which forms on the surface of the fuel. This crust or char layer blocks the input of heat to the unburnt fuel below reducing its burning rate or extinguishing it altogether. Wood tends to exhibit the formation of such char layers. Some additives induce shrinkage of the fuel away from any ignition source whilst others convert the heat input into the evaporation of water of hydration, trapped in a suitable substance such as alumina trihydrate. Intumescent Coatings Another common form of treatment involves the addition of an ‘intumescent’ coating which will swell up under the influence of heat to provide a thermally inert and insulating layer, usually in the form of a paint. These additives can be applied in a number of ways. They can be added as elements of a polymer chain during the polymerisation process or they can be made to attach to an existing material after the polymerisation process. The simplest type of application is the DIY spray-on treatment, but this tends to suffer from the principle that anything which is easy to put on may also be easy to take off. The durability of fire retardant must always be considered by using accelerated ageing or washing before fire testing. Fire retardant chemistry is a complex science and new formulations are continuously being produced by specialist manufacturers. Several of the techniques described above have been successfully applied to reducing the fire hazard of domestic upholstered furniture sold in the UK. Heat release rates have also been significantly reduced. Summary A range .of traditional ‘apparatus bound’ measurement techniques is still widely used throughout the world. The new techniques described here were developed by international collaboration through the International Standards Organisation (ISO). Not only do they provide more useful results, they also offer a route to harmonisation within Europe and ultimately the rest of the world. Two major initiatives are currently under way in Europe, the Construction Products Directive and a directive relating to the fire performance of upholstered furniture. Both involve large programmes of pre-formative research, which are likely to use the measurement techniques described above. |