Apr 12 2017
A recognizable response pattern is produced by conventional electronic noses (eNoses), using a range of dissimilar, but not specific, chemical sensors. Though eNoses have interested developers of artificial intelligence algorithms and neural networks for quite some time, the performance of physical sensors is limited due to overlapping responses and physical instability. In addition, eNoses cannot quantify or separate the chemistry of odors.
zNose®, a new type of electronic nose, uses ultra-fast gas chromatography to simulate an almost-unlimited number of specific virtual chemical sensors and generate olfactory images based on the chemistry of aromas.
Analytical measurements of odors and volatile organic vapors in near real time can be performed by the zNose® with a sensitivity of the order of parts per trillion. Both separation and quantification of separate chemicals within an odor is carried out in a fractions of seconds. Picogram sensitivity, electronically-variable sensitivity and universal non-polar selectivity are achieved using a patented solid-state mass-sensitive detector.
Using the combination of an integrated vapor pre-concentrator and an electronically-variable detector, the instrument can measure vapor concentrations spanning 6+ orders of magnitude. This article shows how a portable zNose® (Figure 1) serves as a useful device for quantifying the concentration of volatile organic compounds in odors emitted by plastic objects.
.jpg)
Figure 1. Portable zNose® incorporated into a handheld instrument
How the zNose® Quantifies the Chemistry of Odors
Figure 2 shows a simplified diagram of the zNose® system, consisting of two parts. One part uses helium gas, a solid-state detector, and a capillary tube (GC column), while the other part comprises of a heated inlet and a pump, which samples ambient air.
A “loop” trap links these two sections, and acts as an injector when placed in the helium section (inject position) and a pre-concentrator when placed in the air section (sample position). The operation follows a two-step process. First, ambient air (odor) is sampled, then organic vapors are collected (pre-concentrated) on the trap. Once sampling is carried out, the trap is subsequently switched into the helium section and the organic compounds that were collected are injected into the helium gas.
These organic compounds travel through a capillary column with varying velocities; hence, individual chemicals exit the column at characteristic times, and while doing so are detected and quantified by a solid-state detector.
.jpg)
Figure 2. Simplified diagram of the zNose® showing an air section on the right and a helium section on the left. A loop trap pre-concentrates organics from ambient air in the sample position and injects them into the helium section when in the inject position.
The collection of sensor data is controlled by an internal high-speed gate array microprocessor, which is transferred to a computer or user interface using an USB or RS-232 connection. The chemistry of odors (Figure 3) can be shown as a polar olfactory image or a sensor spectrum of odor intensity versus retention time.
A single n-alkane vapor standard is used to perform calibration. A repository of retention times of various known chemicals indexed to the n-alkane response (Kovats indices) enables compound identification and machine-independent measurement.
.jpg)
Figure 3. Sensor response to n-alkane vapor standard, here C7-C14, can be displayed as sensor output vs time or its polar equivalent olfactory image
Chemical Analysis (Chromatography)
Shown in Figure 3 is the time derivative of the sensor spectrum, yielding the spectrum of common flux, often known as a chromatogram.
In Figure 4, the chromatogram response of n-alkane vapors (C7 to C14) give an accurate measure of retention times. Graphically-defined regions, shown as red bands in Figure 4, calibrate the system and offer a reference time base against which future chemical responses are indexed or compared. A response midway between C10 and C11, for example, would have a retention time index of 1050.
.jpg)
Figure 4. Chromatogram of n-alkane vapors C6 to C14
Odors from Plastics
Synthetic materials, including rubber, polyester, soft plastics, vinyl, nylon, and rayon and nylon can produce unpleasant odors and may also contain chemicals that can harm humans. Plasticizers including solvents may continue to outgas long after the formation of a plastic object.
For the most part chemicals such as benzene, toluene, 1, 1, 1-trichloroethane, styrene, chloroform, formaldehyde, alcohols and phenol are given off. Plasticizers (phthalates) that remain in the material and do not react after the manufacturing process will outgas and create distinctive odors as well.
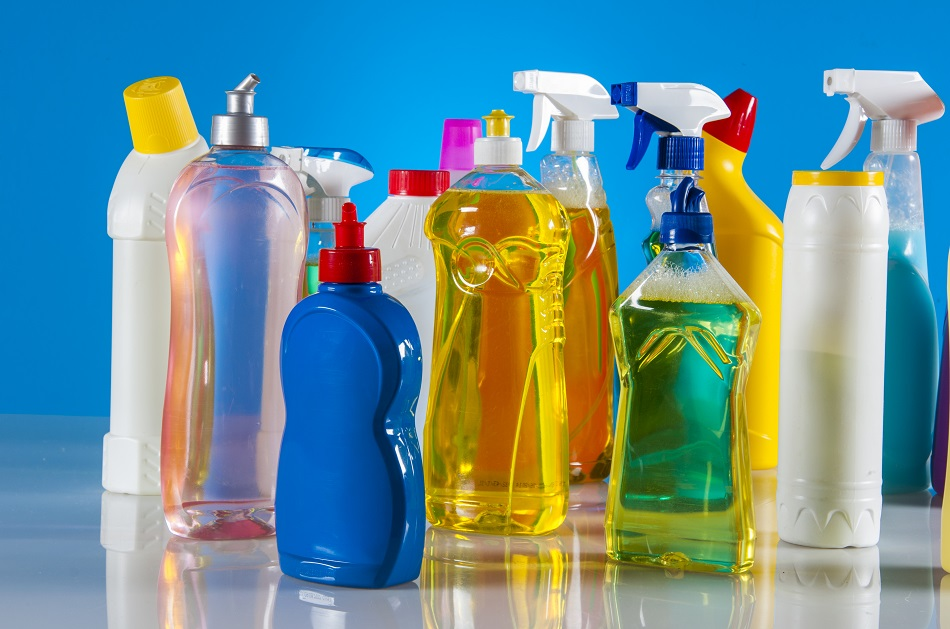
Figure 5. Plastics are a necessary part of the modern environment and serve many useful purposes. Image Credit: FotoSajewicz/Shutterstock.com
Sampling Vapors from Plastics at Room Temperature
Odors and chemical vapors within the vial can be sampled and chemical concentrations can be measured in a controlled and predictable manner using a side-ported sampling needle connected to the inlet of the zNose® (Figure 6). Four plastic rods were divided into two groups of two rods each. Sample no.1 rods produced only a slight odor, while sample no. 2 produced a distinctive and unpleasant odor.
.jpg)
Figure 6. Direct headspace sampling of chemical odors produced from plastic samples placed in a septa-sealed vial
Odors produced by each of the four rods were measured by sampling vial headspace vapors. Approximately 10 mL air was removed from the 40 mL vial through sampling.
Figure 7 shows vertically-offset chromatogram results for each group of sample. Four distinct chemicals were produced by sample 1, while sample 2 produced eight chemicals at much higher concentration measured in peak area (count).
.jpg)
Figure 7. Vapors from samples at 23 °C were evaluated using a 30-second sample time, a 10 °C detector, and a 10ps2a1b method
Testing Sample Vapors at Higher Temperature
Higher vapor concentrations are produced when the temperature of the plastic samples are increased. Measurements taken at such concentrations are more precise than those taken at room temperature.
Figure 8 shows the vial heater which is used to maintain a vial temperature of 60 °C. The temperature of sample vial was maintained for 10 minutes before sampling headspace vapors with the zNose®.
.jpg)
Figure 8. Direct headspace sampling of heated vials
Figure 9 shows the vertically-offset chromatogram results for each sample group. While only six distinct chemicals were produced by sample 1, sample 2 produced nine chemicals at much higher concentrations - measured in peak area (counts).
The presence of extra chemicals, as compared to sample 1, can be clearly seen, and it is presumed that these contribute to the distinctive odor of the plastics in sample 2.
.jpg)
Figure 9. Vapors from samples at 60 °C were evaluated using a <1 minute sample time, a 20 °C detector, and a 10ps2a1b method
Comparing Olfactory Images
Complex odor chemistry can be rapidly identified and compared with the help of high-resolution, two-dimensional olfactory images along with overlaid chromatograms (Figure 10). Plotting can be done for olfactory images using logarithmic odor intensity or linear (radial) odor intensity. These images can be relative or absolute to the highest chemical concentration.
.jpg)
Figure 10. Vaporprint® images together with overlaid chromatograms allow complex odor chemistry to be quickly identified and compared
The derivative of odor intensity gives rise to chromatograms which can then be overlaid for more thorough comparisons. In the above chromatogram, the odor-producing chemicals in sample 2 can be clearly seen. Although these chemicals are not identified by name, they can be tentatively identified by their unique Kovats indices 798, 1000, 1076, 1104, and 1135.
By using only these indices, the zNose® can be employed as a quality control tool to either screen plastic parts for their presence or test plastic production processes.
Conclusion
The zNose® electronic nose enables fast and easy quantification of odor chemistry. Air concentrations well into the parts-per trillion range can now be detected because of the volatility of odor chemicals.
However, headspace measurements are best performed with samples increased to at least 40 °C as volatility is sensitive to temperature. Table 1 shows a summary chart of volatile chemicals by Kovats indices and their intensity in counts.
Table 1. Chemical Composition of Sample Odors
|
Chemical Concentrations (counts) |
Peak 790 |
Peak 933 |
Peak 959 |
Peak 1000 |
Peak 1076 |
Peak 1104 |
Peak 1135 |
Peak 1200 |
Peak 1400 |
Sample 1A |
102 |
659 |
|
93 |
|
261 |
223 |
5509 |
10956 |
Sample 1B |
|
1174 |
|
83 |
|
372 |
91 |
5172 |
8856 |
Sample 2B |
685 |
251 |
116 |
1021 |
1289 |
1053 |
1699 |
11043 |
11398 |
Sample 2B |
401 |
252 |
134 |
1021 |
1451 |
1102 |
2383 |
14321 |
16212 |
Odors from plastics and other synthetic materials can emit unpleasant odors and may also contain chemicals that are hazardous to people. Plasticizers, and solvents, may continue to outgas long after the formation of the plastic object.
Mostly chemicals such as benzene, toluene, styrene, 1, 1, 1-trichloroethane, chloroform, formaldehyde, alcohols, and phenol are produced. Plasticizers (phthalates) that are left in the material (did not react) post the manufacturing process will also outgas and create distinctive odors. The zNose®, using Kovats indices alone, can be employed as a quality control tool to either screen plastic parts for their presence or test plastic production processes.
As a result, odor-causing chemicals can now be rapidly identified and remedial steps can be taken to enhance the quality of plastic materials.
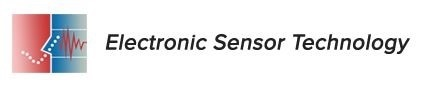
This information has been sourced, reviewed and adapted from materials provided by Electronic Sensor Technology.
For more information on this source, please visit Electronic Sensor Technology.