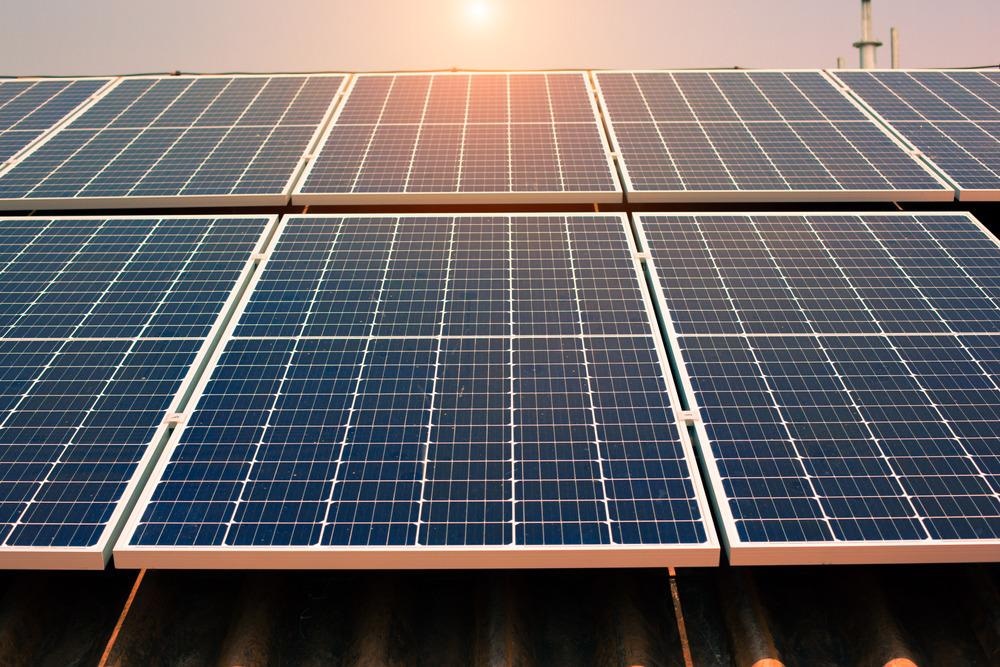
Image Credit: Morakot Kawinchan/Shutterstock.com
Several years of rapid development has allowed research groups worldwide to create increasingly efficient perovskite/silicon tandem solar cells. The scientific focus is now shifting towards the better understanding and control of the mechanisms responsible for the impressive performance of these new types of photovoltaics.
Solar cells based on crystalline silicon have been very successful during the worldwide uptake of photovoltaic (PV) technology and currently dominate the PV market. The vast majority of the commercial solar cells used in solar power plants are of the single-junction type and generate electricity using the single p-n junction.
When sunlight is absorbed in the n-type layer (with a high concentration of electrons), electrons flow towards the p-type layer (with a relatively low concentration of electrons) and creates a useable electrical current.
The maximum efficiency of the laboratory-scale single-junction silicon solar cells has not increased significantly despite the ongoing R&D efforts over the past two decades. The major driving force behind the wide adoption of the PV technology was the economy of scale. It also improved the fabrication process, resulting in low-cost commercial PV modules with sufficiently high power conversion efficiency (PCE).
Fundamental Limitations Restrict the Energy Yield
The PCE of crystalline silicon PV cells has started to saturate at approximately 27% due to the fundamental Shockley–Queisser (SQ) limit and the Auger recombination process.
The SQ limit defines the maximum solar energy conversion efficiency achievable for a particular material (or combination of materials) and is based on the principle of a detailed balance between the photon flux into a PV device to the particle flux (photons or electrons) out of the device and limits the PCE to around 32% for single-junction solar cells.
The PCE is also affected by the Auger recombination process, where the photo-generated charge carriers (electrons and holes) recombine non-radiatively (and transferring energy to other free carriers). This process is an efficiency‐limiting loss mechanism, especially in highly-doped (high carrier density) materials. As a result, the theoretical PCE limit for single-junction solar cells is reduced to approximately 29%.
There are limited prospects to advance the PCE of commercially relevant PV technology by adhering to the incremental approach that has brought the crystalline silicon solar cells to their present dominant position on the PV market.
Future PV Devices Require Innovative Concepts
Multi-junction, or tandem, solar cells consisting of multiple absorber layers with complementary electron bandgaps (the energy difference between the valence band and the conduction band of the material), offer a proven pathway to reach significantly higher performance.
The use of several semiconductor layers with different bandgaps mitigates losses due to charge carrier thermalization (converting the carrier's energy to heat).
In the tandem arrangement, a top PV cell made of a large bandgap material absorbs high-energy photons while permitting lower-energy photons to pass through and be absorbed in the bottom layer PV cell made of smaller bandgap material.
The combined PCE of the tandem cell is enhanced by harvesting higher-energy photons in the top layer and low-energy photons in the bottom layer, surpassing the PCE of a single high- or low-bandgap solar cell.
Tailored Perovskite Materials Boost Tandem Solar Cell Performance
Polycrystalline thin films of metal halide perovskite semiconductors have recently attracted attention as low-cost PV materials due to their solution processability, broad-spectrum light absorption, and low non-radiative recombination losses.
Perovskites are a family of organic-inorganic materials with a crystal structure represented as ABX3 (similar to that of calcium titanate CaTiO3), where A is an organic cation (positively charged molecule), B is a metal cation (typically lead), and X is halogen anion (negatively charged molecule, typically iodine or bromine).
Crucially for tandem solar cell applications, the perovskite bandgap can be tuned by simple substitution of the chemical elements on the A, B, and X sites of the crystal structure, yielding light-absorbing compounds with a range of bandgaps between 1.18 eV and 2.3 eV.
The perovskite-based PV cells are promising candidates for use in tandem solar cells, where they can be paired with the well-established crystalline silicon cells.
Record-Breaking Perovskite/Silicon Solar Cells
Professor Steve Albrecht and his team at Helmholtz-Zentrum Berlin (HZB) in Germany were one of the first to develop and test perovskite/silicon tandem solar cells.
In 2015, Prof. Albrecht's group demonstrated a tandem PV cell with a PCE of more than 18%. In the following years, research groups from the industry and academia joined the R&D efforts after recognizing the tremendous potential of the perovskite/silicon hybrid technology.
At the beginning of 2018, a perovskite/silicon tandem solar cell developed by UK-based clean technology leader and perovskite PV technology pioneer, Oxford PV, demonstrated a PCE of 27%. Later in the year, the company increased the efficiency of its device to 28%.
In 2020, the HZB team demonstrated a record-breaking perovskite/silicon tandem solar cell with an efficiency of 29.15%.
In December 2020, Oxford PV took back the leadership with a PV device showing a PCE of 29.5%.
These efficiency values have been certified by independent laboratories such as the Fraunhofer Institute for Solar Energy Systems (ISE) in Freiburg, Germany, and listed in the chart of National Renewable Energy Laboratory (NREL) in the USA, which tracks the efficiency of nearly all types of PV cells since 1976.
Perovskite-based PV cells entered the chart in 2013. Since then, this class of devices' efficiency has increased much faster than that of the traditional semiconductor-based devices.
The expected practical efficiency limit for perovskite/silicon tandem solar cells is around 35%, and the HZB research team is now aiming to break the 30% efficiency barrier.
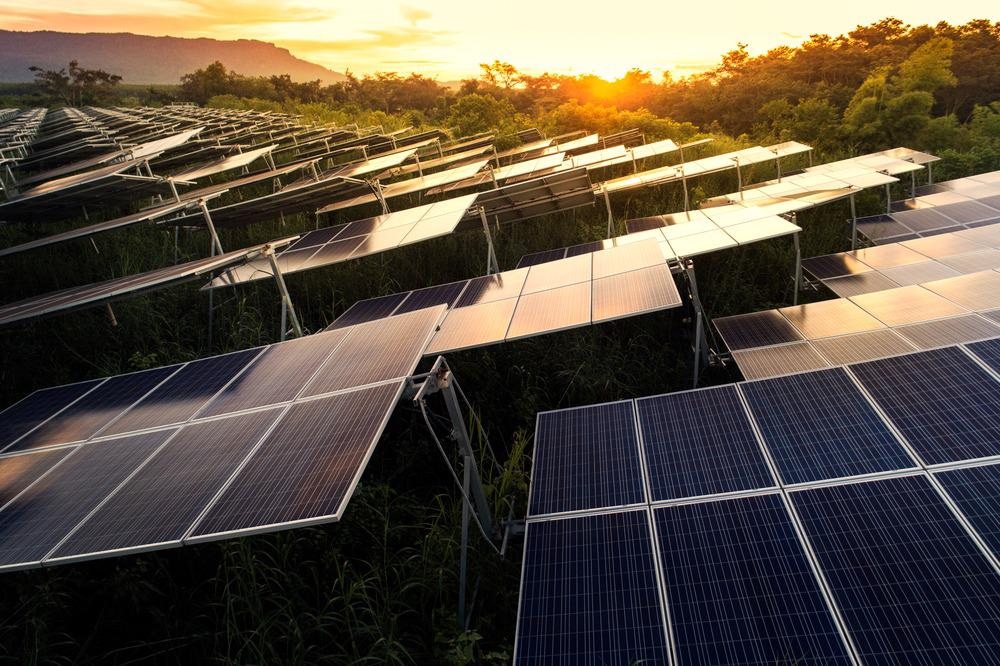
Perovskite/silicon tandem solar cells have an expected efficiency limit of around 35%, with research teams attempting to break the current 30% barrier. Image Credit: Thongsuk Atiwannakul/Shutterstock.com
International Cooperation Is the Key for Success
In collaboration with partners from the Kaunas University of Technology, Lithuania, the University of Potsdam in Germany, the University of Ljubljana in Slovenia, and the University of Sheffield in the UK, Prof. Albrecht's team conducted in-depth studies employing a combination of theoretical modeling, photoluminescence spectroscopy, electrical characterization, and terahertz conductivity measurements to probe the fundamental processes at the interface between the perovskite layer and the underlying crystalline silicon layer.
The detailed investigation allowed the researchers to pinpoint the causes for any significant electrical current losses in the PV device and develop strategies for performance optimization.
In-Depth Characterization Reveals Pathways for Improvement
For the top perovskite semiconducting layer, Prof. Albrecht's team developed a special perovskite formulation containing mixed methylammonium (MA or CH3NH3+) and formamidinium (FA or NH2CH=NH2+) cations in the A site of the compound, and mixed iodine and bromine anions in the X site, resulting in an overall composition of Cs0.05(FA0.77MA0.23)0.95Pb(I0.77Br0.23)3 and a bandgap of 1.68 eV.
According to the researchers' theoretical models, this value ensures optimal distribution of the absorbed solar energy between the perovskite and silicon layers.
The research team found that hole (positive charge carriers) extraction in the device was much slower than electron extraction, limiting the solar cell's PCE.
Optimized Composition and Processing for Greater Efficiency
In collaboration with Lithuania colleagues, Prof. Albrecht's team developed an interfacial layer composed of novel carbazole-based organic molecules with a methyl group substitution (Me-4PACz) that self-assemble in a single-molecule layer at the interface between the silicon and perovskite layers.
This self-assembled monolayer (SAM) serves a twofold purpose: it significantly accelerates hole transport through the device, increasing efficiency while enhancing the contact between the two layers and improving the PV cell's long-term stability. The silicon bottom cell features a particular silicon-oxide top layer for optimal optical coupling to the perovskite top layer.
Testing the stability of their device, the researchers found the tandem solar cell retained 95% of its initial efficiency after 300 hours in ambient air without encapsulation.
All processes utilized to realize the 1 cm2 prototype PV cell are fully scalable and suitable for large-area, easy-to-process photovoltaic devices for future industrial applications.
Improving Long-Term Stability of the Tandem Solar Cells
In parallel, many research groups worldwide work on novel encapsulation methods to protect the perovskite layer from the environment and increase the material's long-term stability.
A research group led by Prof. Anita Ho-Baillie at the University of Sydney, Australia, recently developed an encapsulation process that allowed the perovskite PV cells to pass the strict International Electrotechnical Commission testing standards for heat and humidity for the first time.
The tests demonstrated that the encapsulated perovskite PV cells could withstand outdoor operating conditions with repeated temperature cycling between -40 °C and 85 °C while exposed to 85% relative humidity, bringing the commercial viability of the perovskite/silicon tandem solar cells a step closer.
References and Further Reading
A. Al-Ashouri, et al. (2020) Monolithic perovskite/silicon tandem solar cell with >29% efficiency by enhanced hole extraction. Science, 370 (6522), 1300. Available at: https://doi.org/10.1126/science.abd4016
L. Shi, et al., (2020) Gas chromatography–mass spectrometry analyses of encapsulated stable perovskite solar cells. Science, 368, eaba2412. Available at: https://doi.org/10.1126/science.aba2412
L. McDonald (2020) Lifting the veil on record efficiency: Researchers detail how they created record-setting perovskite/silicon tandem solar cell. [Online] www.ceramics.org Available at: https://ceramics.org/ceramic-tech-today/energy-1/researchers-detail-how-they-created-record-setting-perovskite-silicon-tandem-solar-cell (Accessed on 18 January 2021).
Helmholtz-Zentrum Berlin für Materialien und Energie (2020) Perovskite/silicon tandem solar cells on the magic threshold of 30% efficiency. [Online] www.sciencedaily.com Available at: www.sciencedaily.com/releases/2020/12/201210145817.htm (Accessed on 18 January 2021).
Oxford PV (2020) Oxford PV hits new world record for solar cell. [Online] www.oxfordpv.com Available at: https://www.oxfordpv.com/news/oxford-pv-hits-new-world-record-solar-cell (Accessed on 18 January 2021).
K. A. Bush et al., (2017) 23.6%-efficient monolithic perovskite/silicon tandem solar cells with improved stability. Nature Energy 2, 17009. Available at: https://doi.org/10.1038/nenergy.2017.9
Disclaimer: The views expressed here are those of the author expressed in their private capacity and do not necessarily represent the views of AZoM.com Limited T/A AZoNetwork the owner and operator of this website. This disclaimer forms part of the Terms and conditions of use of this website.