AZoM speaks with Arda Gozen, George and Joan Berry Associate Professor, from Washington State University. Arda was part of a multi-institutional team that has worked to create a scaffold for engineered tissues, by mimicking the properties of human tissue.
Can you give our readers a summary of your recent research?
I'm a manufacturing researcher, so I work on manufacturing processes and machinery. The specific focus that we have had recently, is 3D printing and additive manufacturing with a variety of different materials from polymers to polymer composites and biomaterials.
We're trying to understand how the feedstock material that goes into the 3D printing process and the process parameters, affect the process outcome, as in geometry of the fabricated parts, but more importantly, their functional properties.
We want to discover how we can harness the unique capabilities of 3D printing, understand the fundamental mechanisms that enable such unique capabilities. There's also an applied side to our research, which is wearable/implantable devices.
What does this development mean for the field of tissue replacement?
There's a lot of research going on recently in tissue engineering and every tissue is different, which means engineering of each and every tissue becomes a separate research domain. 3D printing is a common tool that has recently emerged because it can generate heterogeneous structures that mimic the human body and tissues.
The general workflow in 3D printing-enabled tissue engineering is, first, you fabricate the tissue scaffold, either with cells embedded or you introduce the cells later into the scaffold next, the scaffolds go through cell culture during which the cells depending on their type differentiate and proliferate to form a living tissue. During cell culture it is critical that the cells natural environment is replicated as accurately as possible as that will lead to formation of a tissue that mimics the natural tissue the best.
This “environment” involves many factors. As a mechanical engineer, what I understand best are the mechanical aspects of this environment and that is where our contribution lies. Simply, our goal here is to fabricate the scaffolds such that the cells "feel" the same sort of rigidity/softness the actual tissue or actual cells experience in the human body during cell culture. We accomplish this through a new hydrogel formulation and its 3D printing.
People have been using hydrogels for 3D printing purposes, and they're great because they can carry cells, they can carry nutrients with cells, you can 3D print them and then go through cell culture. But those hydrogels are usually quite soft and it is hard to use those standard hydrogels and mimic the properties of stiffer tissues such as cartilage or bones. Even when you think of skin, certain layers are harder than what those conventionally used hydrogels can realize.
What we did here is we combined these three different hydrogels and then we tuned their cross-linking process, and that happened to give us some capability to control the mechanical property in a given range. So if you want to make a structure that is heterogeneous, and you do not want to use very different materials so that you don't go through different compatibility issues, you can use this one single material. As long as you engineer your process carefully, you can generate a mechanical property gradient, which can further your chances of mimicking the native tissues. This combined with some of the capabilities, geometric capabilities of 3D printing, you can actually generate quite different structures, using the same material, so that you can mimic the heterogeneity of tissues.
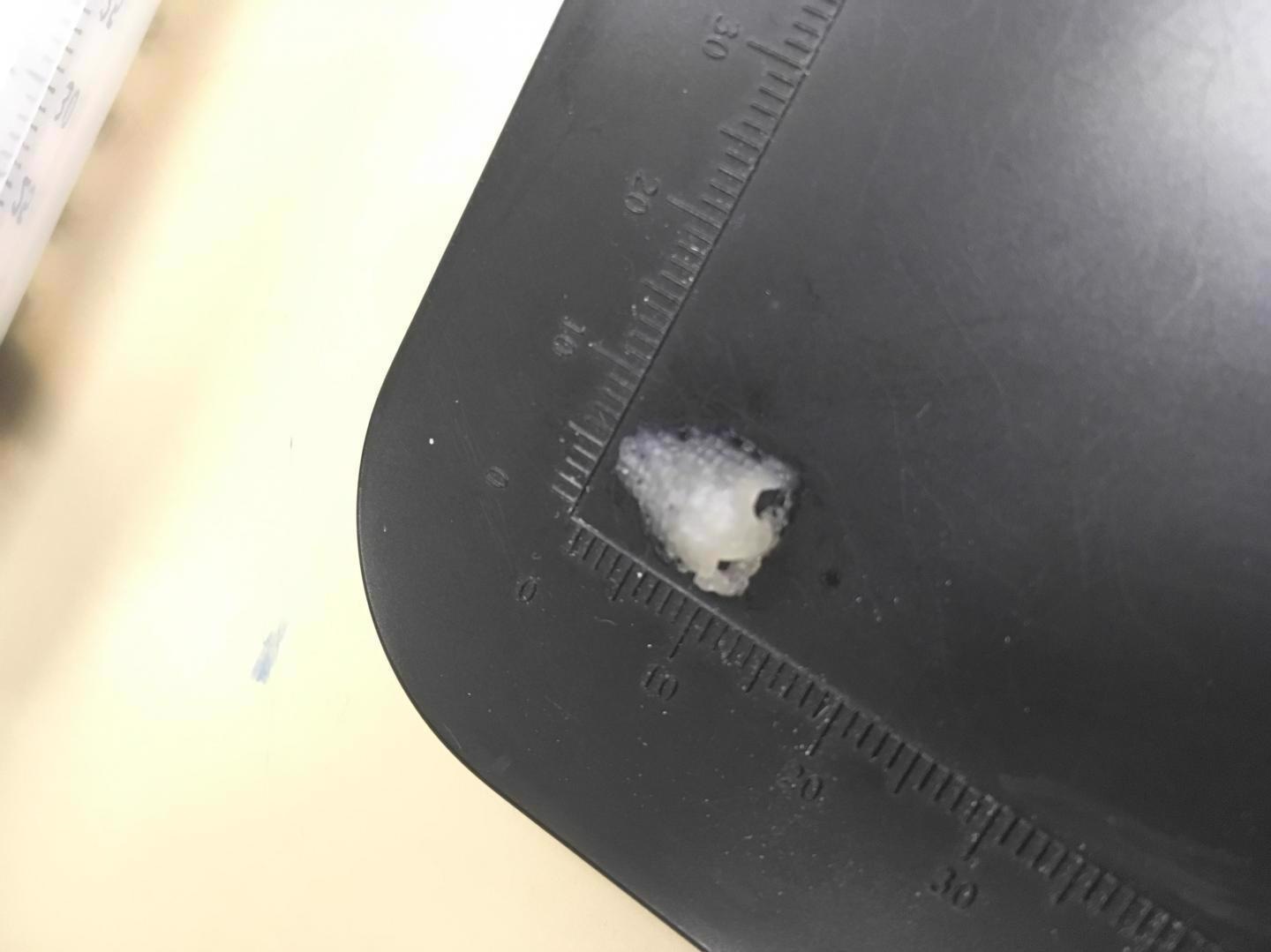
A 3D printed scaffold of a nose. Image Credit: WSU
Can you give our readers more information on the scaffolding material that was used by the team?
We used three commonly used hydrogels and combined them in this new formulation. Hydrogels, in general, are polymers that can hold up, swell up with a lot of water, and hold it for a long time.
One of the hydrogels we used was gelatin, which is heavily used in tissue research. The second material is sodium alginate, maybe the most commonly used hydrogen bio-printing research. And then the third one is gum Arabic. It is actually a food additive that makes things a little bit gummier, as the name implies. There's prior work on, rather than combining three but combining pairs of these materials where you can cross-link them together to generate proper properties for different applications. The credit for the invention of this new hydrogel formulation should go to Dr. Mahmoud Amr, who is the first author of the paper. He very thoroughly studied the literature and ran a bunch of experiments to come up with this idea.
When we combined these hydrogels, we had to consider that each of these hydrogels has their own cross-linking mechanisms. First, we cool the 3D printed constructs because gelatin physically cross-links when it's cold. Next, you go through an ionic cross-linking procedure, which cross-links the sodium alginate and gum Arabic. The final step is the covalent cross-linking of gelatin.
This final step is central to the novelty of this approach. Here, we basically immerse the 3D printed scaffolds in a solution of NHS-EDC for up to 24 hours. Within that 24 hours, the more you keep the construct in there, the stiffer it gets due to increasing cross-linking density. Since the cross-linking process is slow enough, you can stop the process at any time which gives you the opportunity to tune the mechanical properties.
In addition to this capability, the material is quite favorable for 3D printing. We looked into the rheology of the material and found out that the properties of the three hydrogels synergize to realize high resolution 3D printing. Gelatin, for instance, is very viscous at low temperatures, so you cannot really print it. At high temperatures, it becomes really runny, then you cannot really build up layers of material. Sodium alginate also has similar issues. You have to cross-link it for it to maintain its shape, otherwise it is just going to flow and spread out.
When we add gum Arabic, we still need to heat up the material to a high temperature to get a reasonable viscosity to print, but even at those high temperatures, the structure maintains its shape very well. So it gives us a wider window for high resolution 3D printing too. When I say high resolution, we were able to print with nozzles that are smaller than the commonly used nozzles that are utilized in this type of research. That gives us finer feature control too. So it is a bunch of good things coming together in this composite.
How does the method used by your team to mimic natural scaffolds differ from other methods previously used by researchers?
This is a field that is moving so fast, with new material innovations being developed every day. The conventional wisdom for mechanical property control in scaffold fabrication is that when you use more material, your structure becomes more rigid, 3D printed scaffolds are usually made in a porous configuration, so you have these strands going in one way and then the other way, so you have pores in between. When you make them a little tighter, your structure becomes more rigid. Make them a little sparsely placed, they become softer. But by doing that, you are not controlling only one thing.
If these materials are cell loaded, for instance, you are printing cells in there, if you want to make them softer, that means you're putting in less amount of cells in there too. Sometimes these hydrogels are used in an acellular fashion, which is what we do in the paper, just to provide structural support. In that case, if you want to make your structure rigid, for instance, then you want to make those pores really small, if you want to use another material with cells in it, again, in that case, you're running out of cells. So it has coupled effects with cell density. It's not only changing mechanical properties, but you are also hurting some other aspects.
When you think about the material itself being tunable, that means you can keep the pore size constant and just tune the material to make it stiffer, so you don't have to mess with your cell density. So it basically gives you another degree of freedom in controlling mechanical properties and generating gradients that would mimic the human tissues. That would be really the most prominent advantage. The capability of high-resolution 3D printing makes our formulation a very advantageous material.
Are there any benefits of using three different processes to create the scaffold?
By itself, using three different processes does not have any specific advantages. We needed to use those multiple steps because we have a complex material and different materials with different cross-linking requirements. The merit does not lie in using three different processes. Having to use three processes is somewhat of a price you pay, because it makes the process a bit more cumbersome. Having said that, we believe the benefits outweigh the cost. It is more of a process requirement than something we do to gain an advantage.
What's next for the research team?
We are a multi-institutional team. This specific work has been centered at WSU and the team is made up of researchers from WSU, led by myself and Dr. Bernard VanWie, The University of Texas San Antonio, from Dr. Nehal Abu-Lail lab and Morehouse College, from Dr. Juana Mendenhall’s lab. Mahmoud, who was the main author, was from Dr. Abu-Lail’s group with me co-advising him. He just recently graduated. Our team’s main objective is to be able to manufacture articular cartilage that mimics the native tissue.
Specific to this work, I believe we have just scratched the surface in terms of tunability. We are only looking at one composition, for instance, in this paper, because there was so much to study around that composition. We tried some other compositions, they were unsuccessful, but there is still a huge range that we need to look into. Hopefully, that will give us another knob to turn and a wider range of mechanical properties we can achieve.
There are other fundamental things that can be studied that really fall under my interests. For example, how the process parameters affect the mechanical properties. If you print faster or slower or if you use different temperatures, the way the micro-structure of the polymers may vary at the final structure, all of these factors may change mechanical properties. So that can give us another knob to turn in terms of mechanical property control.
This application itself that we demonstrated in the paper was acellular, which means we are not trying to print cells, and if you need to print cells for a given application, obviously it's a little bit more constraining. We print at 50 degrees Celsius, for instance, so we need to go down to physiological temperatures and we have shown that it is possible.
But there are other things to be looked at in terms of cell viability and everything. We had some preliminary data that was put in the revised manuscript that shows that our material is cell-friendly, but there's more work to be done to work with cells, especially if you're going to print the cells.
And finally, the origin of this work, the main motivator was cartilage. This work is born out of an NSF grant that looks into fabricating anatomically accurate articular knee cartilage tissue. I would say the property ranges that we have demonstrated here are not as wide as articular cartilage requires. When you think about the cartilage itself, it is fascinating. Being a mechanical engineer, I was totally oblivious to these facts. Articular cartilage is about a millimeter thick. Within that thin tissue, there are three very distinctly different zones, specifically in terms of mechanical properties. Each zone has anisotropy in different ways. It's just a crazy structure. So we need basically more capabilities from whatever we do to provide mechanical properties to this tissue, such that we can match the wide range of properties with cartilage. So more research towards that is needed, and that is what we're looking into.
Where can our readers find out more information?
Your readers can contact me by email if they have any questions, [email protected]. Additionally, they can access the journal here.
About Arda Gozen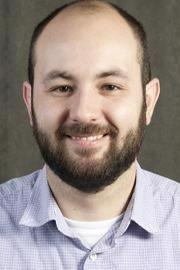
Arda is an associate professor at Washington State University School of Mechanical and Materials Engineering. Arda is currently appointed as a George and Joan Berry associate professor. One of Arda's biggest career highlights is the NSF CAREER grant, awarded in 2018, for his research into 3D printing with polymer nanocomposites.
Arda received the Washington State University Voiland College of Engineering Junior Faculty Research Award in 2019. Currently, Arda runs a lab with five Ph.D. students and 1 MS student, with a majority being women. Arda states, “I'm very proud of this because in mechanical engineering we have a major diversity problem.” Currently, two of Arda’s students have NSF Graduate Research Fellowships, which are very prestigious.
Disclaimer: The views expressed here are those of the interviewee and do not necessarily represent the views of AZoM.com Limited (T/A) AZoNetwork, the owner and operator of this website. This disclaimer forms part of the Terms and Conditions of use of this website.