Interview conducted by Emily Henderson, B.Sc.Oct 6 2021
In this interview, AZoM speaks to Professor Jun Chen about his latest research into bioelectronics, and how he has managed to develop a human-powered bioelectronic that converts human movement into electricity.
What inspired your latest research into bioelectronics?
Since I started my Ph.D. study in 2012 at Georgia Tech, Atlanta, I have become interested in triboelectric nanogenerators and energy-harvesting technology based on materials contact electrification. Unfortunately, this energy-harvesting technology has encountered prevalent challenges such as very low current density and high internal impedance, which arise from their capacitive power generation principle via manipulating the electric dipoles at the materials interfaces.
More importantly, their electrical output performance is vulnerable to ambient humidity caused by sweating and the fluidic environment of the human body, which severely limits their practical on-body applications. An encapsulation layer would enhance the device's humidity resistance; however, it usually compromises extensively the biomechanical-to-electrical energy conversion efficiency. I have spent nearly a decade trying to figure out a way to address these problems.
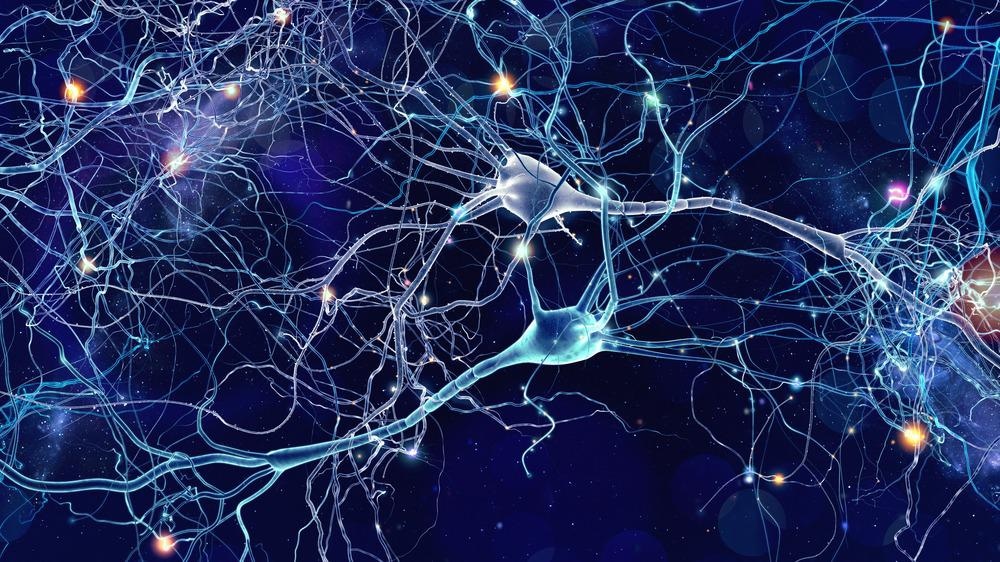
Image Credit: whitehoune/Shutterstock.com
Can you give an overview into what bioelectronics are and some of their applications?
Bioelectronics describes the discipline at the intersection between electronics and living systems. By developing intrinsically waterproof, self-powered bioelectronics, it can have various on-body health care applications, such as a self-powered heart rate monitor or a respiration sensor.
With features like intrinsic humidity-resistance, high current, and low internal resistance, we envision the invented MEG with versatile forms could be widely adopted to build up versatile soft bioelectronics, opening up a new avenue for practical human-body-centered energy, sensing, and therapeutics in the era of Internet of things.
What is the ‘magnetoelastic effect’ and how was this relevant to your latest research?
The magnetoelastic effect – the variation of the magnetic field of a material under mechanical stress – is usually observed in rigid metal alloys such as TbxDy1-xFe2 (Terfenol-D) and GaxFe1-x (Galfenol), with an externally applied magnetic field.
The conventional magnetoelastic effect has been overlooked in the field of bioelectronics for the following three reasons: (1) the rigid metal alloys hold six orders higher mechanical modulus than human bodies, (2) the required mechanical stress for the magnetoelastic alloys is beyond the range of biomechanical stress, and (3) they rely on external magnetic fields resulting in a bulky structure. Our group is the first one in the world to discover the giant magnetoelastic effect in a soft system without the reliance on the externally applied magnetic field.
Why has research into the field of human-powered bioelectronic devices struggled so far?
As previously stated, up until now, existing human-powered bioelectronic devices have not been a practical solution because the optimal mechanical-to-magnetic conversion efficiency in the biomechanical stress range is limited.
In addition, the requirement of the external magnetic field induces structural complexity, and there exists a gigantic mismatch of mechanical modulus (six orders of magnitude difference) between magnetic alloy and human tissue.
Can you describe how you carried out your latest research into designing a human-powered bioelectronic device?
In this work;
- We discovered the giant magnetoelastic effect in a soft system with up to 4 times enhancement of magneto-mechanical coupling compared to what was shown in traditional bulky metal alloys. And it requires no external magnetic field to perform mechanical-to-magnetic conversation, while metal alloy demands an external magnetic field up to 950 Oe via external permanent magnets or bulky electromagnets.
- To fully understand it, we established a wavy chain analytical model based on the magnetic dipole-dipole interaction, which is consistent with the experimental observation.
- To demonstrate its practicability, we took a further step to couple the discovered giant magnetoelastic effect in a soft system with magnetic induction to invent a novel magnetoelastic generator (MEG), which paves a new way for biomechanical-to-electrical energy conversion with unprecedentedly high short-circuit current density and ultralow internal impedance, corresponding to three orders of magnitude improvement than its counterparts in the community.
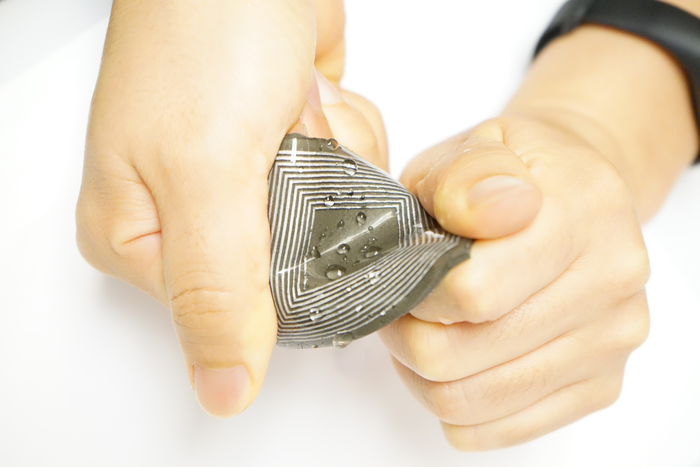
Image Credit: Jun Cheng/UCLA
How does the device convert human body motions, such as bending an elbow, into electricity?
The giant magnetoelastic effect is able to convert stress to localized magnetic field variation, which could be further utilized to generate electricity when coupled with magnetic induction. Thus, a soft MEG was developed with a giant magneto-mechanical coupling (GMMC) layer and a patterned liquid metal receiver as the magnetic induction (MI) layer.
Its mechanism consists of a two-step conversion process: the giant magnetoelastic effect for biomechanical-to-magnetic conversion and the magnetic induction for magnetic-to-electrical conversion. Since the magnetic field variation can pass through the water without a significant intensity decrease, the soft MEG is intrinsically waterproof and could operate stably against heavy perspiration.
What are some of the advantages of having a human-powered device for diagnostic sensors as opposed to traditional bioelectronics?
Bioelectronics are revolutionizing the future of human life by reshaping fields of medicine and healthcare into a more personalized form. Biomechanical-to-electrical energy conversion is a promising pathway to realize self-powered bioelectronics in the imminent era of the Internet of Things.
Do you believe that by having human-powered bioelectronics, we can further advance the field of wearable and implantable diagnostic sensors?
We anticipate that the invention of soft MEGs based on the giant magnetoelastic effect will pave an alternative way for biomechanical energy conversion and open doors to a wide range of possibilities.
What are the next steps for your research into bioelectronics?
We will use novel materials and advanced device design to build up various bioelectronics, which can be used as wearable or implantable power generators and biomedical sensors, opening alternative avenues for human-body-centered applications.
Where can readers find more information?
Paper link: https://www.nature.com/articles/s41563-021-01093-1
UCLA News Report: https://samueli.ucla.edu/ucla-bioengineers-develop-new-class-of-human-powered-bioelectronics/
About Professor Jun Chen
Dr. Jun Chen is currently an assistant professor in the Department of Bioengineering at the UCLA Samueli School of Engineering. His research focuses on nanotechnology and bioelectronics for energy, sensing, and therapeutic applications in the form of smart textiles, wearables, and body-area networks.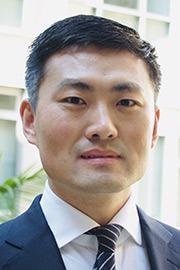
He has published two books, 190 journal articles, with 100 of them being a corresponding author in Chemical Reviews, Chemical Society Reviews, Nature Materials, Nature Electronics, Nature Communications, Science Advances, Joule, Matter, and many others. His works have been highlighted by Nature and Science seven times and covered by global mainstream media more than 1,200 times, including CNN, ABC, NBC, NPR, Newsweek, The Wall Street Journal, Reuters, and Scientific American. He has filed 14 U.S. patents, including one licensed. Dr. Chen has been selected as one of the world’s most influential researchers in the field of materials science by Web of Science.
Among his many accolades are the 30 Life Sciences Leaders To Watch by Informa, UCLA Society of Hellman Fellows Award, Okawa Foundation Research Award, Chem. Soc. Rev. Emerging Investigator Award, Advanced Materials Rising Star, ACS Nano Rising Stars Lectureship Award, IAAM Scientist Medal, 2020 Altmetric Top 100, Top 10 Science Stories of 2020 by Ontario Science Centre, Highly Cited Researchers 2020/2019 in Web of Science, Frontiers in Chemistry Rising Stars, JMCA Emerging Investigator Award, Nanoscale Emerging Investigator Award and the MINE2020 Young Scientist Excellence Award.
More recently, Dr. Chen has been ranked the second most influential researcher in the nanogenerator field globally via an analysis of the bibliometric data, right behind pioneer Prof. Zhong Lin Wang. Beyond research, he is an associate editor of Biosensors and Bioelectronics.
Disclaimer: The views expressed here are those of the interviewee and do not necessarily represent the views of AZoM.com Limited (T/A) AZoNetwork, the owner and operator of this website. This disclaimer forms part of the Terms and Conditions of use of this website.