Many modern electronic devices we use daily owe their existence to the process of semiconductor doping. Doped semiconductors are the result of careful modifications that change pure semiconductor properties.
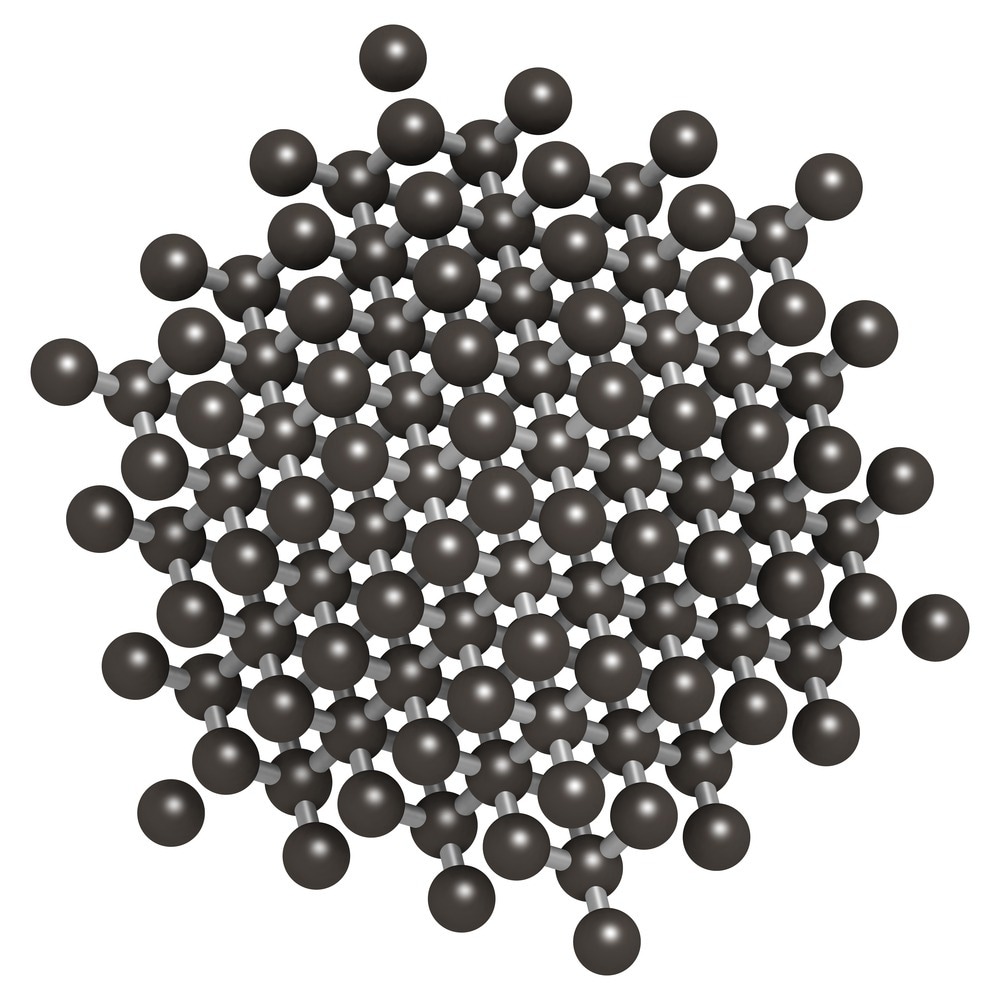
Image Credit: StudioMolekuul/Shutterstock.com
In this article, we will review semiconductor properties, how doping of semiconductors is done, and types of doped semiconductors. We will also provide real-world examples of the use of doped semiconductors in electronic devices and their potential for future technological developments.
Understanding Semiconductors and their Properties
Semiconductors are substances that exhibit electrical conductivities which lie between those of conductors and insulators. They have a bandgap - the energy range where electrons cannot exist - that lies between the valence band (the highest energy level occupied by electrons at absolute zero) and the conduction band (the energy level above the valence band where electrons can move freely).
The energy gap separating the valence and conduction bands is referred to as the bandgap. Pure materials like silicon or germanium are considered intrinsic semiconductors, which possess a valence band filled with electrons and an empty conduction band that is separated from the valence band by a bandgap.
When an electric field is applied to a semiconductor, electrons can be promoted from the valence band to the conduction band, where they can move freely, allowing the material to conduct electricity.
The Mechanisms of Semiconductor Doping
Doping of semiconductors is the process of intentionally adding impurities to a pure semiconductor material to change its electrical properties. The impurities are called dopants, and they can be either a group III element (such as boron, aluminum, or gallium) or a group V element (such as phosphorus, arsenic, or antimony).
When a dopant is added to a semiconductor, it replaces some of the atoms in the crystal lattice, creating new energy levels in the bandgap. These new energy levels, known as dopant states, lie close to the valence or conduction band, depending on the type of dopant used. Doping can result in either p-type or n-type semiconductors.
N-Type Semiconductors
N-type semiconductors are created by doping a semiconductor with an impurity that has more valence electrons than the semiconductor. This creates an extra electron in the semiconductor, which can move freely and conduct electricity. The most common n-type dopant is phosphorus.
P-Type Semiconductors
P-type semiconductors are created by doping a semiconductor with an impurity that has fewer valence electrons than the semiconductor. This creates “holes” in the semiconductor valence band, where electrons can move freely and conduct electricity. The most common p-type doped semiconductor is boron.
The process of doping can be achieved through different methods, such as diffusion or ion implantation. In diffusion, a dopant source, usually in the form of a gas or solid, is introduced to the semiconductor and allowed to diffuse into the material. In ion implantation, dopant ions are accelerated and then implanted into the semiconductor, forming the desired dopant concentration.
Why Dope Semiconductors?
Semiconductor doping has significant practical applications, particularly in electronic devices. For example, p-type and n-type semiconductors are used in the construction of diodes and transistors. Diodes are electrical components that allow current to flow in one direction and not the other, while transistors amplify and switch electronic signals.
Transistors are made from n-type and p-type doped semiconductors and are used in a wide variety of electronic devices, such as computers, radios, and televisions. Solar cells convert sunlight into electricity. They are made from p-type and n-type semiconductors. The type of semiconductor that is used in a solar cell determines how much electricity it can generate.
Light-emitting diodes (LEDs) are another type of electronic device that uses semiconductors. They are able to emit light when an electric current goes through them. LEDs are used in a variety of applications, such as traffic lights, televisions, and computer monitors.
However, the doping of semiconductors also has some potential downsides. For example, if too many dopants are added to a semiconductor, it can become electrically unstable and prone to failure. Additionally, the process of doping can introduce defects into the crystal lattice of the semiconductor, which can affect its mechanical and optical properties.
Doping of semiconductors has revolutionized the electronics industry, enabling the development of smaller, more efficient, and more powerful electronic devices.
The Future of Semiconductor Doping
As technology continues to advance, the demand for more efficient and higher-performing electronic devices will only continue to grow. This will require the development of new doping techniques and the exploration of new materials.
One area of research that shows promise is the use of organic semiconductors, which are composed of carbon-based molecules instead of inorganic materials like silicon. Organic semiconductors have several potential advantages over inorganic semiconductors, including flexibility, low cost, and ease of processing. However, organic semiconductors also pose several challenges, including lower carrier mobility and stability.
Researchers are exploring new doping techniques and materials to address these challenges. For example, one approach is to use a combination of inorganic and organic materials to create hybrid semiconductors that can take advantage of the best properties of both. Another approach is to use new doping techniques, such as plasma doping, which can be used to dope materials that are difficult to dope using traditional methods.
Conclusion
Semiconductor doping is a critical process in the fabrication of semiconductors and has a significant impact on their electrical conductivity. By adding specific impurities to a pure semiconductor material, dopants create new energy levels in the bandgap, altering the number and type of charge carriers and thus affecting the material's conductivity.
The doping of semiconductors has practical applications in the electronics industry, enabling the development of diodes, transistors, and other electronic components. As technology continues to advance, the ability to precisely control the doping process and engineer new semiconductor materials will be essential in the development of even more advanced electronic devices.
More from AZoM: MOSFETs and Their Advantages Over Other Transistors?
References and Further Reading
Abbasi I. (2022). Why and How Do We Dope Semiconductors? [Online], AZoM. Accessible at: URL https://www.azom.com/article.aspx?ArticleID=21657 (accessed 5.13.23).
Chen, F.-C., 2018. Organic Semiconductors, in: Guenther, B.D., Steel, D.G. (Eds.), Encyclopedia of Modern Optics (Second Edition). Elsevier, Oxford, pp. 220–231. https://doi.org/10.1016/B978-0-12-803581-8.09538-2
Enuh B.M. (2023.). Presenting Novel Stable Ultrawide Bandgap Semiconductor Materials [Online]. AZoM. Accessible at: URL https://www.azom.com/article.aspx?ArticleID=22575 (accessed 5.13.23).
Peng, X., Ai, F., Yan, L., Ha, E., Hu, X., He, S., Hu, J., 2021. Synthesis strategies and biomedical applications for doped inorganic semiconductor nanocrystals. Cell Reports Physical Science 2, 100436. https://doi.org/10.1016/j.xcrp.2021.100436
Yoo, H., Heo, K., Ansari, M.H.R., Cho, S., 2021. Recent Advances in Electrical Doping of 2D Semiconductor Materials: Methods, Analyses, and Applications. Nanomaterials 11, 832. https://doi.org/10.3390/nano11040832
Zhao, W., Ding, J., Zou, Y., Di, C., Zhu, D., 2020. Chemical doping of organic semiconductors for thermoelectric applications. Chemical Society Reviews 49, 7210–7228. https://doi.org/10.1039/D0CS00204F
Disclaimer: The views expressed here are those of the author expressed in their private capacity and do not necessarily represent the views of AZoM.com Limited T/A AZoNetwork the owner and operator of this website. This disclaimer forms part of the Terms and conditions of use of this website.