Introduction
The concept of “hybrid organic-inorganic” nanocomposites exploded in the eighty’s with the expansion of soft inorganic chemistry processes [1-4]. Indeed, the various characteristics of the sol-gel process (metallo-organic precursors, organic solvents, low processing temperatures, processing versatility of the colloidal state) allow the mixing of inorganic and organic components at the nanometric scale, in virtually any ratio, leading to the so-called hybrid organic-inorganic nanocomposites [5-18].
Since the development of organic templating growth of inorganic materials in the ninety’s, the elaboration of new types of porous hybrid materials whose structure and function are organized hierarchically is emerging [19]. Ordered periodic micro-, meso- and macroporous materials allow the construction of composites with many guest types, e.g. organic molecules, inorganic ions, semiconductor clusters or polymers. These guest/host materials combine high stability of the inorganic host system, new structure forming mechanisms due to the confinement of guests in well defined pores, and a modular composition. Already in the early studies several proposals were made to apply such ordered porous systems as optical materials and highly specific chemical sensors [20].
Chemical functionalization of the inorganic framework of porous materials, for example through the covalent coupling of an organic moiety, is a promising approach to specific pore surface properties such as hydrophobicity, polarity, and catalytic, optical and electronic activities [21-23]; indeed, the tailoring of the pore surfaces presents direct interest in applications such as catalysis, chromatography, controlled delivery or membranes. Organo-functionalization of ordered mesoporous silica-based solids can be achieved either by post-synthetic methods [24-28] or via direct routes (one-pot synthesis) [29-38]. The latter involves co-condensation of tetraalkoxysilanes and organotrialkoxysilanes in the presence of surfactants. The one-pot synthesis method allows a higher organic content and a more homogeneous organic distribution in the material [39]. Numerous studies have pointed out the role and importance of the nature of the bonds comprising the hybrid inorganic-organic interface [11]. Hybrid materials with covalently bonded organic moieties (Class II hybrids [11]) can be readily designed by sol-gel chemistry and thus a wide range of materials with tailor-made structure and properties can be reproducibly prepared.
In this paper, the preparation strategies of various organically-modified ordered mesoporous silica are reviewed along with some new results. Optically active ordered mesoporous silica materials has been prepared by the covalent coupling of the 2,4-dinitrophenylaminopropyl (DNP) dye to the backbone of an ordered mesoporous MCM-41 silica type material by using the direct grafting process. A classical procedure has been used to form micron-size particles and nanoparticles. An evaporation induce self-assembly process has been adapted to obtain transparent thin films or monoliths. This synthetic route has also been used to produce bimodal mesostructured dye-modified silica materials using both latex spheres and cetyltrimethylammonium bromide (CTAB) micelles as organic structure-directing agents.
Amino and thiol functions have been post-grafted to the backbone of various ordered mesoporous silica materials to produce heavy metal sorbents. In particular, the influence of structure and porosity of the ordered mesostructured silica solids, grafted with various amounts of either aminopropyl or mercaptopropyl moieties, was examined on the access rates of protons, HgII, and CuII to the binding sites. Spherical ordered mesoporous silica micron-size particles functionalized with amino or thiol groups were also prepared via a one-pot synthetic procedure. Mercury adsorption has been performed on both amino and mercapto functionalized silica. Electrochemical techniques have been used to characterize sorption kinetics of Hg(II) in batch reactors and to study the interaction between functionalized silica and mercury(II) at the electrode/solution interface by means of carbon paste electrodes modified with these organic-inorganic hybrids.
Organo-fluorinated mesoporous MCM-41 type silica materials have been prepared by post-synthesis or direct grafting of pentafluorophenyl groups and fluoroalkyl chains. The hydrophobic character of these materials have been evaluated by the measurement of the water amount adsorbed under controlled water-containing atmosphere.
Optically Active Dye-Functionalized Ordered Mesoporous Silica Materials
Preparation of Highly Ordered Mesoporous Dye-Functionalized Silica Micron-Size and Nanometer-Size Particles
Ordered mesoporous silica materials functionalized with 3-(2,4-dinitrophenylamino)-propyltriethoxysilane (TSDP) were made with two different dye molar contents (10 and 20% i.e., 27 and 43 theorical wt%, respectively) by following the experimental procedure reported by Burkett et al. (see Figure 1) [29, 30], tetraethoxysilane (TEOS) being used as additional silica source [40, 41].
The SEM observation shows that ordered dye-functionalized mesoporous silica has precipitated as 5µm-particles with vermicular morphology (see Figure 2).
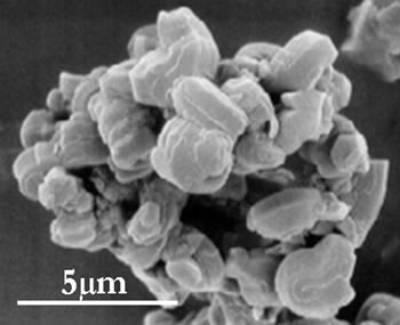
|
Figure 2. SEM micrograph of ordered dye-functionalized mesoporous silica.
|
Small-angle x-ray powder diffraction (SAXRD) patterns (not shown) of the bright yellow as-synthesized materials showed one peak at low angle indicated that they are single phases with mesocopic order. After surfactant extraction, all samples have given a series of high order XRD peaks which have been indexed in the hexagonal symmetry (see Table 1).
Table 1. XRD and N2 adsorption data of surfactant extracted dye-functionalized silica samples.
|
0.9/0.1
|
46.5
|
26.2
|
23.2
|
680
|
24
|
0.89/0.11
|
0.8/0.2
|
43.1
|
25.8
|
22.0
|
390
|
23
|
0.83/0.17
|
Transmission electron microscopy (TEM) images (not shown) of the mesostructured material made with low dye content (x = 0.1) show hexagonal sets of lattice fringes, as well as parallel fringes corresponding to side-on projections of the mesostructure. These observations are consistent with the long range order parameters determined by SAXRD.
Distinct resonances were observed by 29Si Nuclear Magnetic Resonance using Magic Angle Spinning (MAS NMR) for silica (Qn = Si(OSi)n(OH)4-n, n = 2-4) and organosilica (Tm = RSi(OSi)m(OH)3-m, m = 1-3) species. The data indicate that the amount of the dye-functionalized moietie condensed into the wall structure corresponds to that introduced in the synthesis mixture (see Table 1). The 13C CP MAS NMR data qualitatively indicated that 3-(2,4-dinitrophenylamino)-propyl groups were incorporated without significant modification after surfactant extraction.
Nitrogen adsorption studies have confirmed that all dye-functionalized materials after surfactant extraction were predominantly mesoporous (type IV isotherms). The BET surface areas (see Table 1) are less than those typically reported for pure MCM-41 silicas. These reduced specific areas were attributed to pendant chromophore moieties that extend into the channel spaces.
Optical properties of the dye moiety in surfactant extracted organically modified mesostructured silica materials were studied by diffuse reflectance UV-VIS spectroscopy. Two absorption bands were observed at around 350 nm and 440 nm which are characteristic of the chromophore groups [40].
These results suggest that it should be possible to incorporate a range of organic chromophores into ordered mesoporous silicas without extensive modification of their optical properties.
A simple quenching procedure of the template-directed alkaline synthesis of MCM-41 silica leads to the room temperature formation of thermally stable nanoparticles of dye-functionalized mesostructured silica [42].
The experimental conditions were similar to those used previously to prepare hexagonally ordered channel structures for MCM-41 except that the reaction was quenched after 40 seconds by addition of an excess of water, and neutralized with diluted HCl acid to pH 7 after a further 90s or 260s. The resulting dinitrophenylaminopropyl (DNP) functionalized mesostructured nanoparticles were stable as a colloidal suspension for over six months (See Fig. 3). DNP-containing nanoparticles have been prepared with different sizes and mesostructures according to whether the time delay employed between the dilution and neutralization steps was 90 or 260s. Corresponding TEM images of both as-synthesised and surfactant-extracted materials showed either the presence of mesoscopically disordered nanoparticles with a mean diameter of 37 nm (neutralization after 90s), or well-defined particles with hexagonally ordered mesostructured interiors and mean size of 48 nm (neutralization after 260s). Corresponding SAXRD patterns displayed one broad peak at a d-spacing of 6.1 nm (as-synthesized) which then decreased to 5.4 nm upon extraction for the disordered phase, and 4.5 nm (as-synthesized) and 3.7 nm (extracted) for the ordered phase.
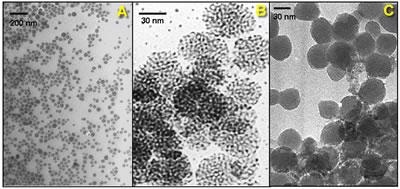
|
Figure 3. TEM images of as-synthesised DNP-functionalised mesostructured silica nanoparticles a) disordered particles, low magnification, b) disordered particles, high magnification c) ordered particles, high magnification.
|
The presence of an intact dye moiety in the mesostructured nanoparticles was confirmed by FTIR and UV-VIS spectroscopic measurements performed on the as-synthesized product.
The data indicated that the DNP functionality was successfully incorporated without degradation, despite the lower rate of hydrolysis of DNPTES compared with TEOS.
The results illustrate not only a potential route to a wide range of functionalized mesostructured nanoparticles but also offer some preliminary insights into the mechanism of growth of such materials.
The covalent attachment of dye molecules into ordered porous MCM-41 type materials could be of general importance in a number of areas such as optics, sensor and membrane technologies. For this reason, it appeared essential to produce mesostructured dye-functionalized silica materials processed as transparent thin films and monoliths.
Mesostructured Dye-Functionalized Silica Processed as Transparent Monoliths
To produce transparent mesostructured DNPTES-modified silica thin films and monoliths (TEOS/DNPTES molar ratio = 0.9/0.1), precursor solutions of the reaction mixture were prepared following the acid synthesis procedure reported by Brinker et al. [43, 44]. Yellow transparent monoliths of several micrometers in thickness have been prepared by slow solvent evaporation from the precursor solution left unstirred in an open teflon dish (see Figure 4a) [41, 45]. Thin transparent films of a few hundred nanometers in thickness were deposited from the same precursor solution by dip-coating on microscope slides at 8 cm.min-1.
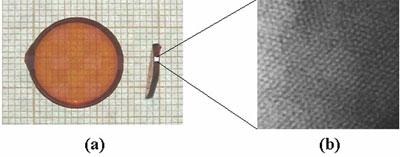
|
Figure 4. (a) Photograph (scale bar = 5mm) and (b) TEM image (scale bar = 10 nm) of the dye-functionalized silica monolith.
|
Small angle XRD patterns recorded on thin films prepared from the above procedure showed two broad peaks at 28.3 and 16.3 Å, that could be assigned to d100 and d110 spacings respectively, indicating hexagonal mesoscopic order in the materials. The monolith exhibited three diffraction lines at 37.0, 21.5 and 17.7 Å, assigned to d100, d110 and d210 spacings respectively, also indicative of long-range hexagonal channel packing. The lower d100 value observed for the thin film materials suggests that smaller surfactant micelles are formed during the relatively rapid drying process associated with the dip-coating method.
TEM images from the monolith sample clearly show hexagonal sets of lattice fringes, as well as parallel fringes corresponding to side-on projections of the mesostructure (see Figure 4b). These observations are in agreement with the long range order parameters determined by XRD.
29Si MAS NMR experiments performed on the monolith samples showed the presence of silica (Qn) and organosilica (Tm) species, and indicated that the amount of organo-functionalized moieties condensed into the wall structures corresponds to that of the synthesis mixture (10 mol% DNPTES). FTIR and 13C CP-MAS NMR spectroscopies indicated that the DNP chromophore was covalently linked into the mesostructured solid as an intact unmodified moiety both before and after surfactant extraction.
Nitrogen adsorption studies of this sample gave a type IV isotherm with a distinct capillary condensation step characteristic of mesoporous solids. A BET specific surface area of 1200 cm2/g and an average BJH pore size of 19 Å were obtained [45].
Optical properties of the mesostructured dye-functionalized materials have been investigated by UV-VIS and fluorescence spectroscopies. Transmission mode was used to perform UV-VIS measurements on thin films deposited on glass slides and monoliths. For all samples, two absorption bands have been observed at around 350 nm and 440 nm which indicate that the optical activity of the dye is retained in thin film and monolith materials. Emission spectra recorded on thin films with an excitation wavelength of 350 nm showed two bands at 495 and 525 nm. The data indicated that the optical activity of the dye moiety was retained when covalently linked to the silica network of the mesostructured material.
Our studies show that the evaporation-induced self assembly process is well adapted to the formation of a continuous mesostructured hybrid siloxane network on a long-range scale [41, 45]. The stable colloidal state involved in this process offers several opportunities in terms of macroscopic shape modeling. In particular, we have used this approach to elaborate dye-functionalized silica materials with bimodal pore size distribution [41, 46].
Dye-Functionalized Silica Material with Bimodal Pore Size Distribution
The synthesis of organically-modified silicas with bimodal pore structures has been investigated [41, 46]. These materials consisted of macropores, surrounded by mesostructured dye-functionalized silica walls. Dual templating methods were employed to control the pore system hierarchy. The macropores are formed by using arrays of monodisperse polystyrene (PS) spheres as templates [47, 48]. The porosity of the wall is controlled by mixing TEOS and DNPTES precursors with cetyltrimethylammonium bromide (CTAB) as the structure-directing agent for the mesostructured dye-functionalized silica walls.
Monodisperse latex beads were ordered into close-packed arrays by slow sedimentation and water evaporation from a colloidal suspension of 140 nm-diameter polystyrene spheres in an open Petri dish at room temperature. Figure 5a shows a scanning electron micrograph of the top layer of the resulting three-dimensional crystalline assembly of polystyrene beads. The 140 nm-diameter polystyrene beads pack into a hexagonal lattice in this layer.
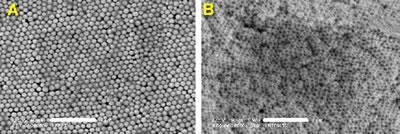
|
Figure 5. (a) scanning electron micrographs of the close-packed array of latex spheres and (b) the hybrid, dye-functionalized silica replica.
|
To produce mesostructured dye-functionalized silica walls around latex beads, the same evaporation-induced self-assembly process in the presence of CTAB mentioned above was employed. The interstices between the latex spheres were permeated by the precursor solution containing pre-hydrolyzed TEOS and DNPTES, and the CTAB surfactant. After complete evaporation of the ethanol, the resulting dry composite solid was stored for 2 days at 50°C to improve the condensation of the siloxane network. Removal of the latex spheres was accomplished by extraction with toluene. Scanning electron micrographs (see Figure 5b) show the dye-functionalized silica replica of several layers of close-packed spheres. This micrograph is consistent with long range order, and monodispersity of the macropore size. An average pore size of 100 nm can be estimated from SEM observations.
The XRD pattern recorded on the material after latex removal showed one broad peak at 39 Å, that indicates that it is a single phase with mesocopic order. The single reflexion XRD pattern observed for the dye-functionalized silica replica suggests that the pore structure is similar to the three dimensional disordered worm-like pore arrays exhibited by MSU type materials [49]. The weak pore ordering is most probably due to the restricted area available for wall formation.
FTIR analysis indicated that the dinitrophenylamino-propyl chromophore was covalently linked into the mesostructured silica network as an intact unmodified moiety. Two absorption bands were observed by UV-VIS diffuse reflectance at around 350 nm and 440 nm which are characteristic of the NO2 chromophore groups and indicated that the optical activity of the dye moiety is retained when covalently linked to the silica network.
Complementary templating strategies have been used to prepare hierarchically ordered dye-functionalized silica materials with bimodal pore size distributions. The strategies are complementary as the two template systems do not destabilize one another.
Amino and Thiol-Functionalized Mesoporous Silica Materials for Heavy Metal Sorbents
Ordered mesoporous silica materials with different pore sizes (about 30-35 Å for small pore size MCM-41 and MCM-48 type materials, and about 60-70 Å for large pore size MCM-41§ and SBA-15 type materials), and spatial pore arrangement (hexagonal for MCM-41 and SBA-15-type materials and cubic for MCM-48-type material) were functionalized with amine and thiol groups by post treatment [50, 51]. Grafting of amine or thiol groups on the walls of MTS samples was performed in dry toluene by reaction with 3-aminopropyltriethoxysilane (APTES) or mercaptopropyltrimethoxysilane (MPTMS). For comparison, not organized silica xerogels with similar pore size (40 and 60 Å, respectively) were also functionalized with amino and thiol groups in the same conditions. Structural characteristics of the resulting aminopropyl-grafted silicas (APS) and mercaptopropyl-grafted silicas (MPS) have been carefully studied. As expected from the space occupied by the organic moieties bound on the inner surface of the mesopores, both specific surface area and pore volume were found to decrease upon modification. This tendency was much more pronounced for small-pore materials and was stronger for samples containing larger amounts of grafted groups. Grafting mesoporous solids displaying small pore size (~35 Å) resulted in great occupation of the pore volume by the organic groups (about 3.1-3.4 mmol cm-3 and 2-2.8 mmol cm-3 for aminopropyl- and mercaptopropyl-groups, respectively), which would affect both accessibility to the active sites and diffusion rates within the hybrid material. Pore volume occupancy in grafted solids with larger pore diameters (SBA-15 and MCM-41§ samples) was found to be 2-3 times lower than in small pore solids. It was checked by XRD measurements that the organized mesoporous architecture remained intact after grafting. Structure collapse was only observed with APS materials because of the basic character of amine groups, which can contribute to local hydrolysis events in the presence of water molecules. This harmful effect was enhanced when increasing the contact time between APS samples and the atmosphere as a result of extended hydration, as highlighted by further decrease in the d100 peaks. Finally, particle size distribution was affected by the grafting process, inducing significant decrease in the average particle size due to attrition occurring during functionalization as a consequence of mechanical stirring. This phenomenon was more important with MPS samples than with APS solids because of longer reaction time in the former case; this behavior was also observed when grafting amorphous silica gels [52].
§: MCM-41 large pore with average size of 60 Å was obtained by adding 1,3,5-trimethybenzene(TMB) acting as CTAB micelle swelling agent (TMB/CTAB molar ratio = 13).
The influence of structure and porosity on the accessibility and the acces rate of H+, HgII, and CuII to the binding sites has been studied to characterize the physical diffusion in these various ordered mesoporous silicas grafted with the same ligands [51]. The ability for a target reactant to reach the organic active sites grafted on the internal surfaces of mesoporous materials has been studied for protonation of APS samples and mercury(II) uptake by protonated APS and MPS solids (see Figure 6). Complexation kinetics have been also characterized for CuII in APS in ethanol.
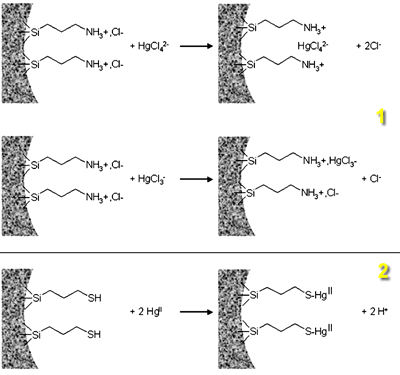
|
Figure 6. Scheme of the binding reaction of Hg(II) to the protonated amine and to the thiol groups; (1) the accumulation of negatively charged chloro-complexes of HgII (HgCl42-and HgCl3-) by electrostatic binding to the protonated amine groups on APS samples, and (2) the preconcentration of HgII species by complexation on thiol groups immobilized on MPS samples.
|
Binding heavy metal species in ordered organosilicas (e.g. HgII in MPS or CuII in APS) was improved in comparison to amorphous silica gels grafted with the same ligands. Accessibility to the binding sites and diffusion rates inside the mesostructure were however affected by the pore size of the starting material as well as the ligand density in the mesopores: (1) 100% accessibility was always observed for large-pore ordered materials (~ 60 Å), while decreasing pore size and increasing ligand population resulted in incompleted filling status because some organic groups remained unavailable to the solution-phase reactants due to steric constraints; (2) the uniform mesoporous structure of the ordered solids imparts to the organic-inorganic hybrids faster kinetics associated to the uptake of metal ion species from diluted solutions, and this effect is markedly more advantageous when using large-pore materials; (3) the advantage of molecular sieve silicas over the amorphous ones disappears when the grafting process is performed in the presence of trace of water (leading to some hierarchical polymerization of the grafting agent in the mesopores), which can lead in this case to superior binding properties for the amorphous materials.
Protonation of aminopropyl groups grafted on porous silica samples is faster in the first half of reaction when using ordered mesoporous solids instead of amorphous materials, but it becomes slower at higher reaction degrees, resulting even in less-than-complete accessibility to the acive sites at equilibrium. These limitations are due to the concentration of charged moieties in a well-structured environment that probably creates strong repulsive electrostatic interactions in such a confined medium, which limits the progression of reactants inside the regular mesopores.
A comparative study was also performed to show the advantage in using grafted silicas with well-ordered three-dimensional structure to modify carbon paste electrodes, which was then applied in voltametric detection after chemical accumulation at open circuit, as compared to similarly grafted amorphous silicas. Mercury(II) was used as a model analyte to characterize the rate of access to the reactive centers within the material and to evaluate the sensitivity of electrodes modified with these organic-inorganic hybrids as a function of their structure. When applied in electrochemical detection of trace Hg(II) after preconcentration, the ordered solids gave rise to voltammetric signals higher by pore than one order of magnitude in comparison to not organized organically-modified silica xerogels.
Better accessibility and lower diffusion resistance in ordered mesoporous organic-inorganic hybrid materials, in comparison to their amorphous counterparts, make them interesting for possible improvements in many applications such as faster remediation processes, lower response time of sensors, greater electrochemical sensitivities, higher catalytic efficiency and turnover.
Ion-exchange abilities of immobilized amine groups towards the chloro-complexes of mercury(II), and on the adsorption of uncomplexed mercury(II) species on the immobilized thiol groups was also studied on spherical ordered mesoporous MCM-41-type silicas, functionalized with aminopropyl (10% APTES molar content) and mercaptopropyl (10% MPTMS molar content) ligands by direct synthesis [53],[54]. MPS material was found to exhibit a very strong interaction with Hg(II). A capacity of 0.6 mmol/g has been measured in batch, corresponding to an accessibility of 60 %. MPS material has been used as model for a new kinetic approach of the mercury adsorption in mesoporous materials. An apparent diffusion coefficient of 2×10-12 cm2/s has been calculated. This material has been incorporated into a carbon paste electrode and the feasibility of the accumulation – electrochemical detection process has been demonstrated. Effect of structure and density of functional groups in these spherical ordered mesoporous silicas directly grafted with mercaptopropyl groups were further investigated by Walcarius et al. [55].
Low MPTMS content (up to 10%) materials present a well-ordered MCM-41 structure over a rather long range, which results in a complete accessibility to all their binding sites; but they underwent some restrictions on mass transport, especially for reaching the active centers located deep in the one-dimensional mesopore channels. Materials displaying less-ordered structures, but still made of cylindrical mesopores organized in a wormhole-like three-dimensional arrangement, were characterized by significantly faster diffusion rates despite their lower pore volumes. The quantitative access to all binding sites was observed up to 30%, while higher functionalization degrees gave rise to less-than-complete filling because of steric hindrance and/or high hydrophobicity. Nevertheless, these adsorbents with high density of functional groups led to very high sorption capacities for HgII species (up to 750 mg.g-1). Materials containing the highest thiol group contents were poorly ordered or even totally disordered and they were subject to dramatic restriction of mass transport rates as well as limited accessibility to the binding sites.
Organo-Fluorinated Ordered Mesoporous Silica Materials
Highly ordered fluorine-containing mesoporous siliceous materials with MCM-41-type architecture were prepared from co-condensation of TEOS and an aromatic fluoro-organosilane precursor, pentafluorophenyltriethoxysilane (PFPTES) or 2-pentafluorophenylethyltriethoxysilane (PFPETES) (See Figure 7) [56]. For materials obtained using precursor PFPTES, the Si-Csp2 bonds were cleaved during the formation and the surfactant extraction processes, leading to fluorine-doped (i.e. F/Si < 0.08) MCM-41 silica materials. However, a better choice of fluoro-organosilane (precursor PFPETES) containing an alkyl spacer between the pentafluorophenyl group and the silicon centre allows to preserve the organic functionality, leading to ordered mesoporous MCM-41-type siliceous materials with higher fluorine contents (F/Si≈0.3).
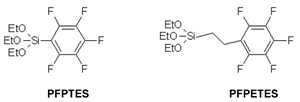
|
Figure 7. Molecular structures of PFPTES and PFPETES organosilane precursors.
|
Further investigations are in due course to improve the fluorine loading, to evaluate the hydrophobic and fluorophilic character of such materials for separation and adsorption applications, and to shape these materials as transparent thin films for optical devices.
Ordered mesoporous MCM-41 type silica materials with average pore size of 20 Å have been post-functionalized with fluoro alkyl chains by using 3,3,3-trifluoropropyltrimethoxysilane (TFPTMS) and 1H,1H,2H,2H-perfluorocetyltriethoxysilane as grafting agents (PFCTES) (See Figure 8) [57]. Ordered mesoporous MCM-41 type silica material functionalized with TFPTMS precursor (10% molar content) was also prepared by a direct-grafting process. Higher organic function molar contents have been measured from 29Si MAS NMR experiments for MCM-41 materials post-grafted with TFPTMS (33% molar content) and PFCTES (13%) [57]. Mainly Q4 units were observed by 29Si MAS NMR in TFPTMS and PFCTES post-grafted materials that characterize a high condensation degree of the silica network. The coexistence of Q3 in a large amount and Q2 units along with Q4 units observed by 29Si MAS NMR in TFPTMS direct-grafted materials indicates the presence of numerous silanol groups.
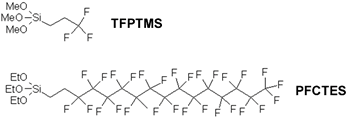
|
Figure 8. Molecular structure of TFPTMS and PFCTES organosilane precursors.
|
The hydrophobic character of all functionalized materials as well as the pure calcined MCM-41 type silica material used for the post-grafting procedures has been evaluated by a isotherm water adsorption test. This latter test consists in the measurement of the weight of water adsorbed by the samples left 48 hours in a controlled water atmosphere with saturated K2SO4 aqueous solution (99% moisture) at a fixed temperature (20°C). Measurements were first made on the dishes used to contain samples. Previous to the incubating period, the samples and the dishes were left one night at 100°C to remove the water adsorbed on the surface. All samples were analyzed by XRD after the isotherm water adsorption test in order to check the structure. Results are reported in Table 2.
Table 2. Organic function content measured by 29Si MAS NMR, water content adsorbed, XRD structure type (after isotherm water adsorption test) of pure calcined MCM-41 silica, post-grafted MCM-41 silicas with TFPTMS and PFCTES, and direct grafted silica with TFPTMS.
|
Starting MCM-41 silica
|
0
|
86.4a
|
MCM-41
|
MCM-41 silica post-grafted TFPTMS
|
33
|
0.6
|
MCM-41
|
MCM-41 silica direct-grafted TFPTMS
|
9
|
55.2
|
disordered
|
MCM-41 silica post-grafted PFCTES
|
13
|
1.8
|
MCM-41
|
a The very high value of water adsorbed measured is probably due to condensed water on the material.
Very low adsorbed water contents were measured on the two post-grafted MCM-41 silicas with TFPTMS and PFCTES (0.6% and 1.8%, respectively) in comparison to the starting MCM-41 silica (86.4%) and the direct grafted MCM-41 silica with TFPTMS (55.4%). The MCM-41 type structure of the TFPTMS direct-grafted silica material collapsed during the water adsorption test. These results indicate a high hydrophobic character for the two post-grafted MCM-41 silicas with TFPTMS and PFCTES for which the MCM-41 type structure was preserved during the water adsorption test. The hydrophilic character of the starting MCM-41 silica and the direct grafted MCM-41 silica with TFPTMS can be related to the presence of a large amount of silanols groups in these two materials. Indeed, 29Si MAS experiments showed that most of the silanol groups present in the starting MCM-41 silica were consumed during the post-grafting process (mainly Q4 units observed for post-grafted materials). A large amount of silanol groups are present in the direct-grafted silica material.
The post-grafting of fluorinated alkyl chains on the pore surface has allowed to make highly hydrophobic ordered mesoporous silica. Such materials present a high potentiel in various application fields such as optics, adsorption and separation.
Conclusion
Our review shows that various organic molecules can be incorported in highly ordered silica material with MCM-41 architecture and modify considerably their properties. Such organically modified materials present a large application potential in areas such as optics, sensing, adsorption, catalysis, separation, environment protection.
The control of the rate of siloxane polymerization and the composite assembly allows to process these mesostructured materials as crack-free, transparent, self-supported films. Such materials could have numerous potential applications in membrane technology and opto-electric devices. The approach applied to elaborate systems with bimodal porosity, is interesting for many applications such as chromatography and catalysis since they combine the faster mass transport of a large pore size system with the high specific surface of a small pore size network. The covalent bonding of organic moieties into the inorganic framework expands their potential use in application fields such as catalysis, selective separations, sensor arrays, wave guides, and miniaturized electronic and magnetic devices. It it is noteworthy that covalent coupling of functional group on the surface of highly ordered mesoporous solids can induce specific functions. As an example, macroscopic effects of second –order optical nonlinearity could be generated from oriented and/or ordered nanostructures of nonlinear agents.
The current investigations emphasize the potential of using hybrid organic-inorganic networks in the synthetic construction of functionalized materials with higher-order structures.
Acknowledgments
Prof. S. Mann, Dr C. E. Fowler, Dr A. Walcarius and Dr G.J.A.A. Soler-Illia are gratefully thanked for their collaboration in these studies.
References
1. H. Schmidt, A. Kaiser, H. Patzelt, H. Sholze, “Mechanical and physical properties of amorphous solids based on (CH3)2SiO-silica gels”, J. Phys., 12 (1982) 275.
2. J. Livage, M. Henry, C. Sanchez, “Sol-gel chemistry of transition metal oxides”, Prog. Solid State Chem., 18 (1988) 259-341.
3. D. Avnir, D. Levy, R. Reisfeld, “The nature of the silica cage as reflected by spectral changes and enhanced photostability of trapped rhodamine 6G”, J. Phys. Chem., 88 (1984) 5956-5959.
4. C. J. Brinker, G. W. Scherrer, “Sol-Gel Science, The Physics and Chemistry of Sol-gel Processing”, Academic Press, San Diego (1990).
5. C. Sanchez and F. Ribot, “Design of hybrid inorganic-organic materials synthesized via sol-gel chemistry”, New J. Chem., 18 (1994) 1007-1047.
6. U. Schubert, N. Hüsing and A. Lorenz, “Hybrid inorganic-organic materials by sol-gel processing of organofunctional metal alkoxides”, Chem. Mater., 7 (1995) 2010-2027.
7. D. A. Loy and K. J. Shea, “Bridged polysilsequioxanes: Highly porous hybrid organic-inorganic materials”, Chem. Rev., 95 (1995) 1431-1442.
8. P. Judenstein and C. Sanchez, “Hybrid organic-inorganic materials : a land of multidisciplinarity”, J. Mater. Chem., 6 (1996) 511-525.
9. R. J. P. Corriu, “Monophasic organic-inorganic hybrid materials”, C.R. Acad. Sci. Paris, Série IIC, 1 (1998) 83-89.
10. C. Sanchez, F. Ribot and B. Lebeau, “Molecular design of hybrid organic-inorganic nanocomposites synthesized via sol-gel chemistry”, J. Mater. Chem., 9 (1999) 35-44.
11. B. K. Coltrain, C. Sanchez, D. W. Schaefer and G. L. Wilkes, “Better Ceramics Through Chemistry VII : Organic/Inorganic Hybrid Materials”, Mater. Res. Soc., Pittsburgh, (1996).
12. R. Laine, C. Sanchez, C. J. Brinker and E. Gianellis, “Hybrid Materials”, Mater. Res. Soc., Pittsburgh, (1998).
13. L. C. Klein, L. F. Francis, M. R. De Guire and J. E. Mark, “Hybrid Materials”, Mater. Res. Soc., Pittsburgh, (1999).
14. R. Laine, C. Sanchez and C. J. Brinker, “Hybrid Materials”, Mater. Res. Soc., Pittsburgh, (2000).
15. E. Bourgeat-Lamy, “Organic-Inorganic Nanostructured Colloids”, J. Nanosci. Nanotech., 2 (2002) 1-24.
16. C. Sanchez, G.J.A.A. Soler-Illia, F. Ribot, C.R. Mayer, V. Cabuil, T. Lalot, “Designed Hybrid Organic-Inorganic Nanocomposites from Functional Nanobuilding Blocks”, Chem. Mater., 13 (2001) 3061-3083, and references therein.
17. C. Sanchez, G.J.A.A. Soler-Illia, F. Ribot and D. Grosso, “Design of functional nano-structured materials through the use of controlled hybrid organic–inorganic interfaces”, C. R. Chimie, 6 (2003) 1131-1151, and references therein.
18. P. Gomez-Romero and C. Sanchez, “Functional Hybrid Materials”, Wiley-VCH, Weinheim (2003).
19. G.J.A.A. Soler-Illia, C. Sanchez, B. Lebeau and J. Patarin, “Chemical Strategies To Design Textured Materials: from Microporous and Mesoporous Oxides to Nanonetworks and Hierarchical Structures”, Chem. Rev., 102 (2002) 4093-4138, and references therein.
20. C. Sanchez, B. Lebeau, F. Chaput and J.-P. Boilot, “Optical Properties of Functional Hybrid Organic-Inorganic Nanocomposites”, Adv. Mater., 15 (2003) 1969-1994.
21. K. Moller and T. Bein, “Inclusion Chemistry in Periodic Mesoporous Hosts”, Chem. Mater., 10 (1998) 2950-2963.
22. A. Stein, B. J. Melde, R. C. Schroden, “Hybrid Inorganic-Organic Mesoporous Silicates - Nanoscopic Reactors Coming of Age”, Adv. Mater., 12 (2000) 1403-1419.
23. A. Sayari and S. Hamoudi, “Periodic Mesoporous Silica-Based Organic-Inorganic Nanocomposite Materials”, Chem. Mater., 13 (2001) 3151-3168.
24. R. Burch, N. Cruise, D. Gleeson and S. C. Tsang, “Surface-grafted manganese–oxo species on the walls of MCM-41 channels-a novel oxidation catalyst”, Chem. Commun., (1996) 951-952.
25. T. Maschmeyer, F. Rey, G. Sankar and J. M. Thomas, “Heterogeneous catalysts obtained by grafting metallocene complexes onto mesoporous silica”, Nature, 378 (1995) 159-162.
26. D. Brunel, A. Cauvel, F. Fajula and F. Di Renzo, “MCM-41 type silicas as supports for immobilized catalysts”, Stud. Surf. Sci. Catal., 97 (1995) 173.
27. D. Brunel, “Functionalized micelle-templated silicas (MTS) and their use as catalysts for fine chemicals”, Micropor. Mesopor. Mater., 27 (1999) 329-344.
28. D. Brunel, A. C. Blanc, A. Galarneau and F. Fajula, “New trends in the design of supported catalysts on mesoporous silicas and their applications in fine chemicals”, Catal. Today, 73 (2002) 139-152.
29. B. Burkett, S. D. Sims and S. Mann, “Synthesis of hybrid inorganic–organic mesoporous silica by co-condensation of siloxane and organosiloxane precursors”, Chem. Commun., (1996) 1367-1368.
30. C. E. Fowler, S. L. Burkett and S. Mann, “Synthesis and characterization of ordered organo-silica-surfactant mesophases with functionalized MCM-41-type architecture”, Chem. Commun., (1997) 1769-1770.
31. D. J. MacQuarrie, “Direct preparation of organically modified MCM-type materials. Preparation and characterisation of aminopropyl–MCM and 2-cyanoethyl–MCM”, Chem. Commun., (1996) 1961-1962.
32. M. H. Lim, C. F. Blanford and A. Stein, “Synthesis and Characterization of a Reactive Vinyl-Functionalized MCM-41: Probing the Internal Pore Structure by a Bromination Reaction”, J. Am. Chem. Soc., 119 (1997) 4090-4091.
33. F. Babonneau, L. Leite and S. Fontlupt, “Structural characterization of organically-modified porous silicates synthesized using CTA+ surfactant and acidic conditions”, J. Mater. Chem., 9 (1999) 175-178.
34. L. Mercier and T. J. Pinnavaia, “Direct Synthesis of Hybrid Organic-Inorganic Nanoporous Silica by a Neutral Amine Assembly Route: Structure-Function Control by Stoichiometric Incorporation of Organosiloxane Molecules”, Chem. Mater., 12 (2000) 188-196.
35. Y. Lu , H. Fan, N. Doke, D. A. Loy, R. A. Assink, D. A. Lavan, C. J. Brinker, “Evaporation-Induced Self-Assembly of Hybrid Bridged Silsesquioxane Film and Particulate Mesophases with Integral Organic Functionality”, J. Am. Chem. Soc., 122 (2000) 5258-5261.
36. T. Asefa T. , M.J. Maclachlan, N. Coombs, G.A. Ozin, “Periodic mesoporous organosilicas with organic groups inside the channel walls”, Nature, 402 (1999) 867-871.
37. S. Inagaki, G. Shiyou, T. Ohsuna, O. Terasaki, “An ordered mesoporous organosilica hybrid material with a crystal-like wall structure”, Nature, 416 (2002) 304-307.
38. B. J. Melde, B. T. Holland, C. F. Blanford and A. Stein, “Mesoporous Sieves with Unified Hybrid Inorganic/Organic Frameworks”, Chem. Mater., 11 (1999) 3302-3308.
39. M. H. Lim and A. Stein, “Comparative Studies of Grafting and Direct Syntheses of Inorganic-Organic Hybrid Mesoporous Materials”, Chem. Mater., 11 (1999) 3285-3295.
40. C. F. Fowler , B. Lebeau and S. Mann, “Covalent coupling of an organic chromophore into functionalized MCM-41 mesophases by template-directed co-condensation”, Chem. Commun., (1998) 1825-1826.
41. B. Lebeau, C.E. Fowler and S. Mann, “Tailoring of organically-modified silica MCM-41 type Material with Optically Active Molecule”, «Better Ceramics Through Chemistry VIII : Organic-Inorganic Hybrid Materials», (Ed. R. Laine, C. Sanchez and C. J. Brinker), Mater. Res. Soc., Pittsburgh, 628 (2000) cc4.1-cc4.12
42. C. E. Fowler. D. Kushalani, B. Lebeau and S. Mann, “Nanoscale Materials with Mesostructured Interiors”, Adv. Mater., 13 (2001) 649-652.
43. Y. Lu, R. Ganguli, C. E. Drewlen, M. T. Anderson, C. J. Brinker, W. Gong, Y. Guo, H. Soyez, B. Dunn, M. H. Huang and J. I. Zink, “Continuous Formation of Supported Cubic and Hexagonal Mesoporous Films by Sol–gel Dip-coating”, Nature, 389 (1997) 364-368.
44. C. J.Brinker, Y. Lu, A. Sellinger and H. Fan, “Evaporation-Induced Self-Assembly: Nanostructures Made Easy”, Adv. Mater., 11 (1999)
45. B. Lebeau, C.E. Fowler, S.R. Hall and S. Mann, “Transparent Thin Films and Monoliths Prepared from Dye-functionalized Ordered Silica Mesostructures”, J. Mater. Chem., 9 (1999) 2279-2281.
46. B. Lebeau, C. E. Fowler, S. Mann, C. Farcet, B. Charleux and C. Sanchez, “Synthesis of Hierarchically Ordered Dye-functionalised Mesoporous silica with Macroporous Architecture by Dual Templating”, J. Mater. Chem., 10 (2000) 2105-2108.
47. O. D. Velev, T. A. Jede, R. F. Lobo and A. M. Lenhoff, „Porous Silica via Colloidal Crystallization“, Nature, 389 (1997) 447-448.
48. B. T. Holland and C. F. Blanford, A. Stein, “Synthesis of Macroporous Minerals with Highly Ordered Three-Dimensional Arrays of Spheroidal Voids”, Science, 281 (1998) 538-540.
49. S. A. Bagshaw, E. Prouzet and T. J. Pinnavaia, “Templating of Mesoporous Molecular Sieves by Nonionic Polyethylene Oxide Surfactants”, Science, 269 (1995) 1242-1244.
50. A. Walcarius, M. Etienne, S. Sayen and B. Lebeau, “Grafted Silicas in Electroanalysis : Amorphous Versus Crystalline Mesoporous Materials”, Electroanalysis, 15 (2003) 414-421.
51. A. Walcarius, M. Etienne and B. Lebeau, “Rate of Access to the Binding Sites in Organically Modified Silicates – Part 2. Ordered Mesoporous Silicas Grafted with Amine or Thiol Groups”, Chem Mater., 15 (2003) 2161-2173.
52. A. Walcarius, M. Etienne and J. Bessière, “Rate of Access to the Binding Sites in Organically Modified Silicates. 1. Amorphous Silica Gels Grafted with Amine or Thiol Group”, Chem. Mater., 14 (2002) 2757.
53. M. Etienne, B. Lebeau and A. Walcarius, “Organically-modified Mesoporous Silica Spheres with MCM-41 Architecture”, New J. Chem., 26 (2002) 384-386.
54. M. Etienne, S. Sayen, A. Walcarius and B. Lebeau, “Organically-modified Mesoporous Silicate Spheres with MCM-41 Architecture as Sorbents for Heavy Metals”, Stud. Surf. Sci. Catal., (Ed. A. Sayari and M. Jaroniec), Elsevier Science, 141 (2002) 615-622.
55. A. Walcarius and C. Delacôte, “Rate of Access to the Binding Sites in Organically Modified Silicates. 3. Effect of Structure and Density of Functional Groups in Mesoporous Solids Obtained by the Co-Condensation Route”, Chem. Mater., 15 (2003) 4181-4192.
56. B. Lebeau, C. Marichal, A. Mirjol, G. J. A. A. Soler-Illia, R. Buestrich, M. Popall, L. Mazerolles and C. Sanchez, “Synthesis of Highly Ordered Mesoporous Hybrid Silica from Aromatic Fluorinated Organosilane Precursors”, New J. Chem., 27 (2003) 166-171.
57. F. Le Berrurier, C. Marichal and B. Lebeau, unpublished results.
Contact Details
|