Introduction
The design of highly efficient photocatalytic systems which work for the reduction of global atmospheric pollution and the purification of polluted water is attractive and one of the most desirable yet challenging goals in the research of environmentally-friendly catalysts [1-8]. TiO2 photocatalysts have been attracting much attention in resent years, because TiO2 photocatalyst can completely mineralize toxic and nonbiodegradable organics to CO2, H2O, and inorganic constituents [3]. Many research have been undertaken to use TiO2 photocatalyst for purification of polluted water. During the process of purification of polluted water, photocatalyst has to be separated from the treated water. To separate the photocatalyst from water, photocatalyst should be supported on bulk materials. As photocatalyst support, SiO2 and zeolite has been widely used [1, 3-7]. On the other hand, silicon carbide (SiC) has physical bulk properties such as high thermostabilty, high mechanical strength, and high electrical conductivity and has been used as catalyst support [8-12]. In addition, SiC is easily molded into a filter and has been already used for exhaust emission filter of automobile [10]. Although it may be a useful support for photocatalysts used in liquid phase, there have been no reports on the properties of TiO2 photocatalyst deposited on SiC. In the present study, we have dealt with the preparation and characterization of TiO2 photocatalysts deposited on SiC support using the TiC-SiC nano particle precursor synthesized by the carbothermic reduction method [13] and demonstrated the successful utilization of this TiO2-SiC catalyst for the photocatalytic degradation of 2-propanol diluted in water.
Experimental Procedure
To synthesize TiC-SiC precursor, titanium tetraisopropoxide (TPOT), tetraethyl orthosilicate (TEOS), and phenolic resin were mixed at room temperature. After the continuous stirring of the mixtures to the gelation, the gels obtained were pyrolyzed at 1273 K under a nitrogen atmosphere. This material was preheated at 1873 K under Ar flow to yield the TiC-SiC precursor by the carbothermic reduction [13]. The obtained TiC-SiC powders (Ti/Si=0.4 in molar ratio) were then calcined in air at 873 K to synthesize TiO2-SiC photocatalyst. TiO2-SiO2 photocatalyst (Ti/Si=0.4 in molar ratio) were prepared by the sol-gel method using TEOS, TTIP and ethanol and calcined in air at 873 K. The X-ray Absorption Near Edge Structure (XANES) spectra were measured at the BL-7C facility of the Photon Factory at the National Laboratory for High-Energy Physics, Tsukuba. X-ray diffraction patterns of the samples were measured by Rigaku RINT2500 diffractometer with CuKα radiation. X-ray photoelectron spectroscopy (XPS) was recorded with JEOL microprobe system using the Mg Kα line. The photocatalyst (50 mg) was transferred to a quartz cell with an aqueous solution of 2-propanol (2.6x10-3 mol dm-3, 25 ml). Prior to UV-light irradiation, the suspension was stirred in a flow of O2 for 1 h under dark conditions. The sample was then irradiated at 295 K using UV light (λ> 250 nm) from a 100 W high-pressure Hg lamp with continuous stirring under O2 atmosphere in the system. The products were analyzed by gas chromatography. Water adsorption isotherms of the catalysts was measured at 293 K using a conventional vacuum system.
Results and Discussion
Figure 1 shows the XRD patterns of TiC-SiC precursor and TiO2-SiC photocatalyst prepared from TiC-SiC precursor and TiO2-SiO2 photocatalyst prepared by the sol-gel method and calcined at 873 K. In the TiC-SiC sample, very sharp XRD peaks assignable to TiC and SiC were observed, indicating well-crystallized TiC-SiC precursor was obtained. After calcination at 873 K, anatase and rutile phases from the crystalline TiO2 were observed on TiO2-SiC photocatalyst. However, TiO2-SiO2 photocatalyst calcined at 873 K exhibited no XRD peaks due to the crystallized phases, indicating that the TiO2 species exist in an amorphous phase or as ultrafine particles. TiO2-SiO2 photocatalyst calcined at 1173-1473 K exhibited the XRD peaks of anatase but the crystallinity was lower than that of TiO2-SiC photocatalyst calcined at the same temperature and the surface area decreased significantly at the high calcination temperature, surface area changed from 169 m2g-1 at 873 K to 0.6 m2g-1 at 1473 K.
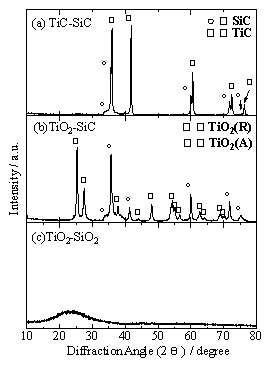
Figure 1. XRD patterns of TiC-SiC precursor (a), TiO2-SiC photocatalyst (b), and TiO2-SiO2 photocatalyst (c).
The XANES spectra at Ti K-edge of TiO2-SiC photocatalyst and TiO2-SiO2 photocatalyst are shown in Figure 2. The XANES spectra at the Ti K-edge show several well-defined pre-edge peaks which are related to the local structure surrounding the Ti atom. The relative intensities of these pre-edge peaks provide useful information on the coordination number of the Ti atom [1]. TiO2-SiC photocatalyst exhibited three small well-defined pre-edge peaks which can be assigned to a presence of a mixture of anatase and rutile with high crystallinity. However, TiO2-SiO2 photocatalyst exhibit an intense single pre-edge peak, indicating that part of titanium oxide species have a tetragonal coordination in the SiO2 matrix [5].
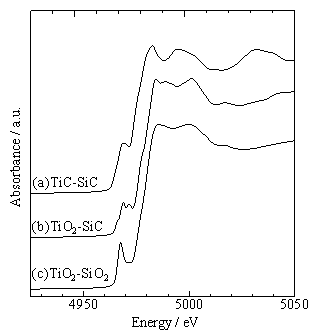
Figure 2. Ti K-edge XANES spectra of TiC-SiC precusor (a), TiO2-SiC photocatalyst (b), and TiO2-SiO2 photocatalyst.
Figure 3 shows the Ti 2p and Si 2p XPS spectra of TiO2-SiC photocatalyst and TiO2-SiO2 photocatalyst. The position of Ti 2p XPS band indicated that TiO2 existed on the surface of both photocatalysts. Comparing TiO2-SiC with TiO2-SiO2, peak intensity corresponding to Ti 2p3/2 and Ti 2p1/2 on TiO2-SiC was higher than that on TiO2-SiO2. This result indicates that the content of TiO2 on TiO2-SiC surface is higher than that on TiO2-SiO2 surface and most part of TiO2 species of TiO2-SiO2 exists inside of SiO2 matrix. Si 2p spectrum revealed that SiC on the surface of TiO2-SiC was partially oxidized into SiO2 by the calcination of TiC-SiC.
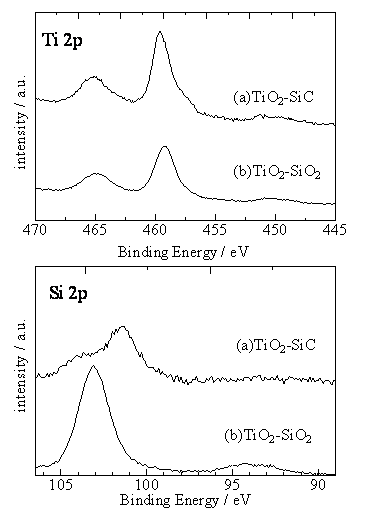
Figure 3. Ti 2p and Si 2p XPS spectra of TiO2-SiC photocatalyst (a) and TiO2-SiO2 photocatalyst (b).
To investigate the photocatalytic reactivity of TiO2-SiC photocatalyst, degradation of 2-propanol diluted in water was carried out under UV-light irradiation. Figure 4 shows the reaction time profiles of the liquid-phase photocatalytic reaction on the TiC-SiC precursor, TiO2-SiC photocatalyst, and TiO2-SiO2 photocatalyst. When UV-light turned on, photocatalyst decomposed 2-propanol into acetone, CO2, and H2O, and finally, acetone was also decomposed into CO2 and H2O. On the other hand, TiC-SiC precursor did not decompose to 2-propanol. TiO2-SiC photocatalyst decomposed 2-propanol faster than TiO2-SiO2 photocatalyst. This indicates that SiC is efficient support for nano-sized TiO2 photocatalyst which can be utilized for degradation of organic compounds in water.
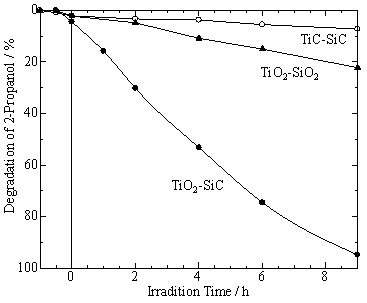
Figure 4. Photocatalytic degradation of 2-propanol diluted in water on TiC-SiC precursor, TiO2-SiC photocatalyst, and TiO2-SiO2 photocatalyst.
Figure 5 shows the photocatalytic reaction rate normalized by weight of TiO2 included within catalysts after 6 h irradiation on TiO2-SiC photocatalyst, TiO2-SiO2 photocatalyst, and the amounts of water adsorption on TiO2-SiC photocatalyst and TiO2-SiO2 photocatalyst are also shown. TiO2-SiC photocatalyst exhibited the higher photocatalytic reactivity than TiO2-SiO2 photocatalyst and the amount of water adsorption on TiO2-SiC photocatalyst is much smaller than on TiO2-SiO2 photocatalyst. The hydrophobic property of TiO2-SiC photocatalyst may be caused by the hydrophobic property of SiC surface. This indicates that well-crystallized TiO2 deposited on SiC has an efficient photocatalytic reactivity and hydrophobic surface of SiC support is also very important factor to realize the efficient photocatalytic reactivity for the liquid phase reaction.
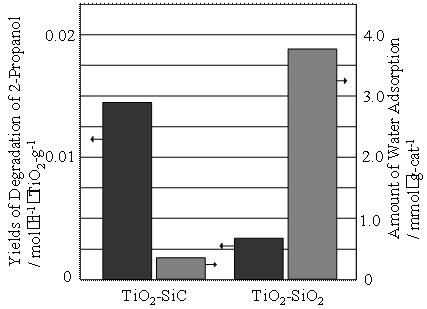
Figure 5. Photocatalytic reactivity for 2-propanol and amount of water adsorption on TiO2-SiC photocatalyst and TiO2-SiO2 photocatalyst.
Conclusions
TiO2 photocatalysts deposited on SiC (TiO2-SiC) prepared by the calcination of the TiC-SiC precursor showed high photocatalytic reactivity for the degradation of 2-propanol diluted in water. TiO2-SiC photocatalyst showed the higher photocatalytic reactivity than the TiO2-SiO2 photocatalyst prepared by the conventional sol-gel method. The formation of well-crystallized TiO2 on SiC and the hydrophobic surface of SiC support were found to be related to the efficient photocatalytic reactivity of TiO2-SiC photocatalyst. Since SiC is mechanically strong enough to be used as a filter for water purification, SiC is a good candidate as support for TiO2 photocatalysts used in liquid phase reactions.
Acknowledgements
The present work is supported by the Grant-in-Aid for Scientific Research (KAKENHI) in Priority Area “Molecular Nano Dynamics” from Ministry of Education, Culture, Sports, Science and Technology (No. 17034036, No. 17360388). This work is partly performed under the project of collaborative research at the Joining and Welding Research Institute (JWRI) of Osaka University. The X-ray adsorption experiments were performed at the Photon Factory of KEK (2004G295).
References
1. H. Yamashita and M. Anpo, ”Local Structures and Photocatalytic Reactivities of the Titaniumoxide and Chromium Oxide Species Incorporated within Micro- andmesoporous Zeolite Materials: XAFS and Photoluminescence Studies”, Current Opinion in Solid State & Maters. Sci., 7, 471-459 (2003).
2. N. Takeda, T. Torimoto, S. Sampath, S. Kuwabata and H. Yoneyama, “Effect of Inert Supports for Titanium Dioxide Loading on Enhancement of Photodecomposition Rate of Gaseous Propionaldehyde”, J. Phys. Chem., 99, 9986-9991 (1995).
3. T. Ishikawa, H. Yamaoka, Y. Harada, T. Fujii and T. Nagasawa, “A General Process for In situ Formation of Fundamental Surface Layers on Ceramics”, Nature, 416, 64-67 (2002).
4. H. Yamashita, Y. Ichihashi, M. Harada, G. Stewart, M.A. Fox and M. Anpo, “Photocatalytic Degradation of 1-Octanol on Anchored Titanium Oxide and on TiO2 Powder Catalysts”, J. Catal., 158, 97-101 (1996).
5. H. Yamashita, S. Kawasaki, Y. Ichihashi, M. Harada, M. Anpo, G. Stewart, M. A. Fox, C. Louis and M. Che, “Characterization of Titanium-Silicon Binary Oxide Catalysts Prepared by the Sol-Gel Method and Their Photocatalytic Reactivity for the Liquid-Phase Oxidation of 1-Octanol”, J. Phys. Chem. B, 102, 5870-5875 (1998).
6. H. Yamashita, K. Maekawa, H. Nakao and M. Anpo, “Efficient Adsorption and Photocatalytic Degradation of Organic Pollutants Diluted in Water using Fluoride-modified Hydrophobic Mesoporous Silica”, Appl. Surf. Sci., 237, 393-397 (2004).
7. K. Ikeue, H.Yamashita, T. Takewaki and M. Anpo, “Photocatalytic Reduction of CO2 with H2O on Ti-βZeolite Photocatalysts: Effect of the Hydrophobic and Hydrophilic Properties”, J. Phys. Chem. B, 105, 8350-8355 (2001).
8. H. Yamashita, K. Maekawa, Y. Nakatani, J.-J. Park and M. Anpo, ”Photocatalytic Degradation of 2-Propanol Dilluted in Water with TiO2 Photocatalyst loaded on Si3N4”, Chem. Lett., 32, 910. (2003)
9. Z. Liu, W. Shen, W. Bu, H. Chen, Z. Hua, L. Zhang, L. Li, J. Shi and S. Tan, “Low-temperature Formation of Nanocrystalline β-SiC with High Surface Area and Mesoporosity via Reaction of Mesoporous Carbon and Silicon Powder”, Micropor. Mesopor. Mater., 82, 137-145 (2005).
10. K. Guerfi, S. Lagerge, M.J. Meriziani, Y. Nedellec and G. Chauveteau, “Influence of the Oxidation on the Surface Properties of Silicon Carbide”, Thermochim. Acta, 434, 140 (2005).
11. N. Keller, C. Pham-Huu, M. J. Leduox, C. Estournes and G. Ehret, “Preparation and Characterization of SiC Microtubes”, J. Appl. Catal. A-Gen., 187, 255-268 (1999).
12. N. Keller, O. Reiff, V. Keller and M. Ledoux, “High Surface Area Submicrometer-sized β-SiC Particles Grown by Shape Memory Synthesis Method ”, Diam. Relat. Mater., 14, 1353-1360 (2005).
13. M. Narisawa, H. Ukon, K. Okamura, S. Shimada and T. Katayama, “Synthesis of SiC-TiC Powders by Pyrolysis of Phenolic Resin-Alkoxides Hybrid Precursors”, J. Ceram. Soc. Jan., 110, 518-522 (2002).
Contact Details
|