It may be difficult to appreciate, as you tap laboriously away at the keyboard of your laptop, that the materials of the critical electronic joints are experiencing conditions which are just as, if not more, severe than those suffered by their cousins in power generation plant. While the operating temperatures may be widely different (up to 1000K), the common denominator in terms of material behaviour is the homologous temperature, Th, which is the ratio of the temperature to the melting temperature (in K). Values of Th in either application may be similar, figure 1. 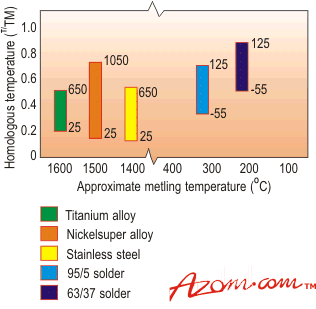 | Figure 1. Homologous temperature ranges for engineering alloys in their typical high temperature applications. | Driving Force for Development The usual means of interconnection in electronic devices is the soldered joint, the technology for which has evolved slowly over centuries from its use in traditional joining applications. Alloy selection has been determined primarily by processing performance (wettability, flowability etc) rather than the intrinsic properties of the solder itself. Since about the mid-80’s, there have been tremendous developments in electronics and the majority of these have been associated with ever increasing demands on the materials involved. The requirements for miniaturisation, faster switching and the emergence of surface mounted technology, in which joints must play a structural as well as electrical role, have resulted in the conditions in the laptop, (or even more acutely in automobile or aerospace electronics) being on a par with those in the gas turbine engine. The situation has not stabilised and will continue as long as the competitive edge provided by enhanced performance remains. With literally hundreds of thousands of connections in large assemblies, sometimes dire consequences of failure, a growing demand for reliability and longevity as the products mature, some universal questions emerge. As for all high temperature components. These include: • Is it possible to predict lifetime more accurately and reliably? • Can design be improved to ensure longer life? • Can similar improvements be achieved by using better materials? • And in the case of electronics can toxic lead be eliminated from solders? There is considerable international effort devoted to these questions, notably in the USA - much less overtly in the UK. A popular strategy is to adopt the fairly established methodologies for steam generating plant and gas turbines to the soldered joint case. Categories of Solder While literally hundreds of various solders exist, there are three broad categories: eutectic or near eutectic, tin lead alloys (63:37 wt%); high lead alloys (≈95%Pb-Sn); and others. While this last group contains many more specialist alloys, including the sought after lead free solders, our knowledge and understanding overwhelmingly resides with the first two types and these will be the focus of present attention. Eutectic Alloys Eutectic alloys possess the lowest melting point of the tin lead system (and hence operate at the highest homologous temperature). Their microstructure comprises colonies of alternating lamellae of α and β phases under equilibrium conditions. An electronic joint is a miniature casting which may be produced under a wide range of cooling rates. As these increase the colony size and lamella spacing decrease, and under quite rapid cooling rates a spheroidal a phase structure results. Lead Rich Solders Lead rich solders, containing between 90 and 97.5% lead are used for higher temperature applications. In equilibrium at room temperature they contain a two-phase structure that reverts to a single-phase solid solution on heating to temperatures above 100°C. The equilibrium microstructure of both alloy groups is intrinsically unstable and coarsens during storage, even at room temperature. This instability is accentuated by strain and cyclic strain that may have significant implications on service performance. Surface Mount Technology On traditional printed circuit boards (PCBs) the integrated circuit appears as a dual inline package and the solder is attached into the holes in the board. Surface mount technology (SMT) uses smaller components that are soldered directly onto the pad surface of the board. Smaller holes are required and the components may be mounted on either side of the board. The solder acts as the mechanical attachment, the electrical connector and often the avenue for heat dissipation. It is predicted that SMT will constitute some 80% of all components by the year 2000. Schematic examples of common joint geometries used for attaching components to boards are shown in figure 2. With the trend towards greater interfacial areas a layered structure may be used to accommodate thermal strain. Leadless (i.e. without leads, not lead, Pb) are most vulnerable to this problem because of their intrinsic structural rigidity. 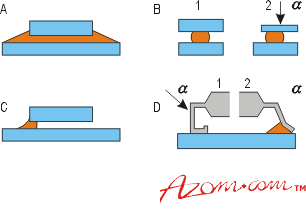 | Figure 2. Common joint geometries, (a) die or substrate bonding, (b) solder bump, (c) leadless chip carrier and (c) leaded chip carrier. | An additional metallurgical factor arises when the overall joint is considered. This is the formation of intermetallics such as Cu3Sn, Cu6Sn5 or Au-Sn, which constitutes the bonding process. These are brittle compounds that are able to grow during service and may profoundly affect the strength of the joint. Service Conditions In service, soldered joints experience thermal fluctuations and, to a much lesser extent, mechanical vibrations. The former arise from internal heat generation during on/off cycling and/or from changes in the operating environment which in extreme cases, such as automobiles or aircraft may range from around -50°C to 125°C. These conditions are at least equivalent to those experienced by nickel-based superalloys in gas turbines, figure 1. Thermal Expansion Since the materials of the individual components in the joint possess markedly different coefficients of thermal expansion, mismatch strains, often of the order of 10%, develop which assume the cyclic nature imposed by the operating conditions, figure 3. Damage accumulates until failure occurs under thermomechanical fatigue, usually in shear since the solder is essentially a soft layer. Unfortunately for the designer, there is a further factor to be taken into account. The time dependency of both the mechanical behaviour per se, and the instability of the microstructure. Again the high homologous temperature is responsible, and the degree of microstructural instability exceeds that for steels, titanium or nickel alloys in their common applications at elevated temperature. 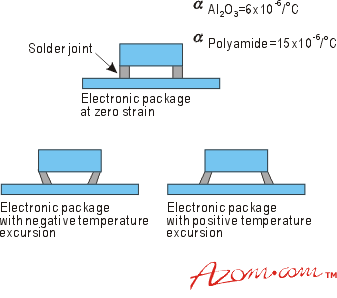 | Figure 3.Development of strains with temperature change (where α is the co-efficient of thermal expansion) | At room temperature solders are ‘mushy’, exhibiting elastic, anelastic and viscoplastic behaviour under the action of monotonic stress. Published values for Young's modulus vary wildly due to the difficulty in maintaining truly elastic conditions. High strain rates and small stresses are essential to eradicate time dependent effects. Rapidly cooled microstructures tend to be slightly stronger than those produced by slow cooling but it has been suggested that such differences disappear after storage at room temperature. Superplastic behaviour is exhibited with the appropriate combination of grain size, temperature and strain rate. Creep Hold periods in the service cycle allow either creep (under stress controlled conditions) or, more commonly, stress relaxation (under strain limited conditions) to occur. The majority of available creep data for solders refers to minimum strain rates that are unrealistically high (>10-4 s-1) relative to those likely in service. A sigmoidal relationship exists between applied stress and minimum creep rate indicating a shifting dominance in deformation mechanism. Again, microstructural alteration, or prior strain, may have a significant (≈threefold) effect on creep behaviour. While stress relaxation and creep are usually regarded as being mechanistically equivalent, the stress exponent in the stress/strain rate expression is usually slightly greater (by approximately 1) in the former. Fatigue Behaviour Under high strain fatigue (i.e. when the plastic component of the total strain exceeds the elastic contribution normally the case for solders). Solders follow the Coffin Manson expression: Nfβ Δεp = C Where Nf is the number of cycles to failure, Δεp the plastic strain range and β and C are constants. At small strain ranges (less than about 1%), β increases giving a bilinear relationship. Perusal of data from different sources requires considerable caution particularly with regard to the definition of failure employed. Significant variations may arise from tests performed in tension/compression or shear, using load drop or electrical continuity as indicators of failure. The fatigue behaviour of eutectic solders tends to be relatively insensitive to temperature over the likely operating range of 50°C to 150°C. Lead rich solders are more affected exhibiting a reduced endurance with increasing temperature up to 150°C. As for most metals, there is a critical frequency (3 x 10-4 Hz at 35°C and 3 x 10-3 Hz at 150°C for eutectic alloys), below which further reduction in frequency (more strictly strain rate) has a deleterious effect on life. There is some debate concerning the effect of prior ageing on fatigue endurance that centres around whether the ageing process obliterates the initial microstructure. Fatigue damage in eutectic solders is usually concentrated into recrystallised and coarsened bands leading to the destruction of the colony structure and its replacement by equiaxed α and β phases. As with the classic precipitate strengthened aluminium alloys, the coarse soft bands localise subsequent deformation until crack initiation occurs. At low strain ranges intergranular cracking tends to be dominant which is also the predominant cyclic failure mechanism in lead rich solders. Moving closer to service experience, the continuous cycling responses described above should be amplified by a consideration of hold, or dwell, effects. Both lead tin solder groups have been found to be tensile dwell sensitive, i.e. a considerable life debit (in excess of an order of magnitude) as compared with continuous cycling, is produced by incorporation of a dwell at maximum tensile strain in each cycle. Cycles with similar dwells in compression, or dwells in both tension and compression, have little influence on endurance, figure 4. The reduction in life increases with increasing duration of the tensile dwell until saturation is observed for hold periods above a few minutes. Mechanistically, this behaviour is attributed to conversion of the stress relaxation during the hold period to grain boundary damage. 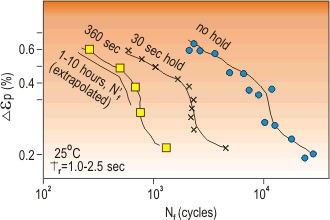 | Figure 4. Effect of tensile dwells on fatigue endurance. | In actual service, the principal fluctuations in strain are themselves generated by variations in temperature, resulting in failure by thermomechanical fatigue. So, over the range of temperatures involved the behaviour of materials may be quite different. During a single cycle the extent of time dependent effects may vary considerably according to the location of the dwell in the cycle. For example, it has been estimated that at 80°C stress relaxation is complete in about one minute whereas at -55°C it will be incomplete after several days. Similar comments apply to microstructural changes arising from the inherent instability of solders. While it is relatively easy to thermally cycle entire joint configurations, detailed information regarding stress and strain values and their distribution is usually lacking, and the results are restrictively specific to the particular geometry under examination. Conversely, thermomechanically fatigue testing to produce generically valuable material data is very expensive. It has been found that when cycling a lead rich solder between zero and a tensile strain, hold periods at either extreme were damaging to life. Cycling between 20°C and 80°C produced a shorter life than isothermal fatigue at 80°C particularly under small strain rates. In direct contrast to isothermal fatigue, endurance was diminished by higher frequencies, and minimum life resulted from cycling strain and temperature in phase but with a 90°C separation. Performance Prediction At present, prediction of the performance of solder presents more problems than, say, for steels, nickel or titanium alloys. This is due to the usually higher homologous temperature, the greater instability and inhomogeneity and the relative scarcity of data upon which valid comparisons may be made. To estimate joint performance, of course, requires accurate stress analysis in addition to reliable and representative materials data. The small and often irregular shape of joints adds a further complication. Predictive Methodologies With regard to material behaviour, in the USA considerable effort has been devoted to evaluating the applicability of predictive methodologies used for materials in steam and nuclear power plant and gas turbines, to solders. There has been limited success to date for many reasons such as: • insufficient data are available for strain range partitioning • fracture mechanics approaches are thwarted by the onset of general yielding • the difficulty encountered in determining crack lengths. Integration of damage rate equations has been fruitful but even here there is an implicit assumption that the microstructural instability does not produce a change in dominant failure mode. There is a pressing need to gain better insight into the mechanisms of failure, and how they are influenced by potential service conditions, so that life prediction methods can have a more secure foundation. Fundamental Deficiencies of Solders Current lead tin solders suffer two fundamental deficiencies, one technical and one environmental. The former is the intrinsic vulnerability to cyclic deformation whereby localisation of damage results in low endurance. Microstructural engineering to produce small equiaxed grains and a homogeneous structure by thermomechanical processing, alloy or particle addition offer solutions in principle, but the level of understanding of the microstructural response to the stress, strain, time, temperature profiles encountered in service is inadequate to pursue this route with total confidence. Further, it would need to be ascertained that potential solutions to the fatigue problem did not impair processability of solders, which is of paramount importance. The second, and potentially more dangerous problem faced by lead tin solders is the lead itself. As a toxic metal, it is under growing pressure from the environmental lobby for its elimination. Given the constraints imposed by processability, toxicity, availability and cost, the number of options available for the replacement of lead containing solders is not large. Tin appears to be the ideal candidate for the basis of the new generation of solders and alloys containing ~3wt% silver or 0.7wt% copper seem the most promising. These rankings have been reached mainly upon processing criteria - the entire gamut of mechanical testing, necessary to ensure structural integrity, has yet to be undertaken. Nonetheless, there is little doubt that within our lifetime lead-tin solders will be defunct. The prize for those first in the race to replace them will be enormous, while the also rans might follow lead! Summary The ever-increasing demands for improved performance and the continued push for further miniaturisation of electronic devices is focusing attention on the ability of solder to provide structural integrity. Since the consequences of joint failure may be potentially as catastrophic as with more conventional large scale structures, the comparative lack of progress in validating design is disturbing. The tension between mechanical conservatism in design and performance requirements is increasing rapidly. In the medium term, it seems inevitable that conventional lead containing solders will be replaced by their more environmentally friendly equivalents. Even when the prime candidates have been selected and appraised in terms of processability, the problem of structural integrity will remain. It is timely for materials engineers to anticipate this situation and commence their investigations now since we are in a void of knowledge and understanding on this aspect. |