Apr 23 2019
Human skeletal muscles are soft yet strong, resistant to fatigue, and full of water—a special combination of properties that are sought after by materials researchers for their own creations.
A mechanically-trained artificial muscle resists damage (crack) propagation using aligned nanofibrils, a similar fatigue-resistant mechanism as in skeleton muscles. (Image credit: Ji Liu, Shaoting Lin, and Xinyue Liu)
Now, a new research performed by researchers at MIT has revealed that this total package of properties can be imparted to synthetic hydrogels by subjecting them to a vigorous workout.
The researchers, in particular, mechanically trained the synthetic hydrogels by expanding them in a water bath. And the repetitions at the “gym” paid off just as with skeletal muscles. The workout aligned nanofibers within the synthetic hydrogels to create a soft, robust, and hydrated material that is resistant to fatigue or breakdown over a countless number of repetitive movements.
The polyvinyl alcohol, or PVA, hydrogels trained in the experiment are popular biomaterials that are utilized by scientists for drug coatings, medical implants, and many other applications, informed Xuanhe Zhao, an associate professor of mechanical engineering at MIT. “But one with these four important properties has not been designed or manufactured until now.”
Zhao and his colleagues have described in their paper, which was recently published in the Proceedings of the National Academy of Sciences, how the hydrogels can also be 3D-printed into a wide range of shapes that can be trained to form the set of muscle-like characteristics.
In the coming days, the materials can possibly be utilized in implants like “heart valves, cartilage replacements, and spinal disks, as well as in engineering applications such as soft robots,” stated Zhao.
Postdoc Ji Liu, graduate student Shaoting Lin, and graduate student Xunyue Liu in Zhao’s lab are other MIT authors on the paper.
Training for strength and more
Heart valves and muscles are examples of excellent load-bearing natural tissues that are a bioinspiration to materials scientists; however, designing materials that are capable of capturing all their characteristics at the same time has been very difficult, stated Zhao.
For example, a hydrogel can be designed using highly aligned fibers to add strength to it; however, it may not have the required water content to make it compatible for use in humans, or it may not be as supple as a muscle.
Most of the tissues in the human body contain about 70 percent water, so if we want to implant a biomaterial in the body, a higher water content is more desirable for many applications in the body.
Xuanhe Zhao, Associate Professor, Department of Mechanical Engineering, MIT
The finding that a muscle-like hydrogel could be produced through mechanical training was something of a coincidence, stated Lin, the lead author of the PNAS study. The scientists had been conducting cyclic mechanical loading tests on the hydrogels, in an attempt to locate the fatigue point where the hydrogels would start to break down. Instead, they were surprised to find that the hydrogels were, in fact, being strengthened by the cyclic training.
The phenomenon of strengthening in hydrogels after cyclic loading is counterintuitive to the current understanding on fatigue fracture in hydrogels, but shares the similarity with the mechanism of muscle strengthening after training.
Shaoting Lin, Graduate Student, Department of Mechanical Engineering, MIT
Prior to training, the nanofibers making up the hydrogel are arbitrarily oriented.
“During the training process, what we realized is that we were aligning the nanofibers,” stated Lin, and added that the alignment is akin to what occurs to a human muscle under frequent exercise. A training like that rendered the hydrogels fatigue-resistant and stronger. The combination of the four major characteristics appeared after approximately 1,000 stretching cycles, yet certain hydrogels were expanded more than 30,000 cycles without breaking down. The trained hydrogel’s tensile strength—in the orientation of the aligned fibers—increased by roughly 4.3 times compared to the unstretched hydrogel. Simultaneously, the hydrogel exhibited soft flexibility, and sustained a high water content of 84%, discovered the team.
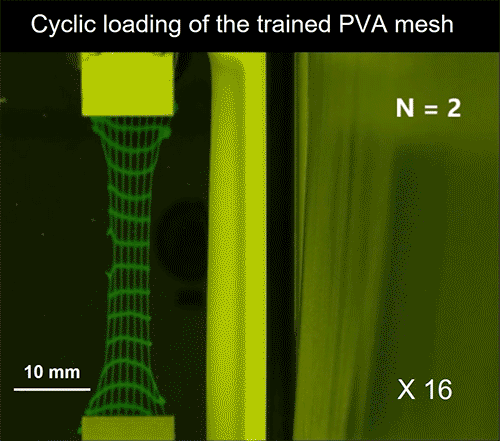
Video credit: MIT
The antifatigue factor
The researchers eventually turned to confocal microscopy to closely examine the trained hydrogels, to check whether they can possibly find out the reasons behind their remarkable anti-fatigue trait.
We put these through thousands of cycles of load, so why doesn’t it fail? What we did is make a cut perpendicular to these nanofibers and tried to propagate a crack or damage in this material.
Shaoting Lin, Graduate Student, Department of Mechanical Engineering, MIT
We dyed the fibers under the microscope to see how they deformed as a result of the cut, [and found that] a phenomenon called crack pinning was responsible for fatigue resistance.
Ji Liu, Postdoctoral Research Associate, Department of Mechanical Engineering, MIT
In an amorphous hydrogel, where the polymer chains are randomly aligned, it doesn’t take too much energy for damage to spread through the gel. But in the aligned fibers of the hydrogel, a crack perpendicular to the fibers is ‘pinned’ in place and prevented from lengthening because it takes much more energy to fracture through the aligned fibers one by one.
Shaoting Lin, Graduate Student, Department of Mechanical Engineering, MIT
As a matter of fact, the trained hydrogels break a well-known fatigue threshold, projected by the Lake-Thomas theory, which proposes the energy needed to fracture one layer of amorphous polymer chains like those making up the PVA hydrogels. Zhao and his colleagues concluded that the trained hydrogels are 10 to 100 times more fatigue-resistant than projected by the theory.
The study was partly supported by the National Science Foundation, the Office of Naval Research, and the U.S. Army Research Office through the Institute for Soldier Nanotechnologies at MIT.