By Surbhi JainReviewed by Susha Cheriyedath, M.Sc.Apr 20 2022
In an article recently published in the journal ACS Applied Energy Materials, researchers presented insights on the air stability and surface chemistry of the storage of garnet solid electrolytes.
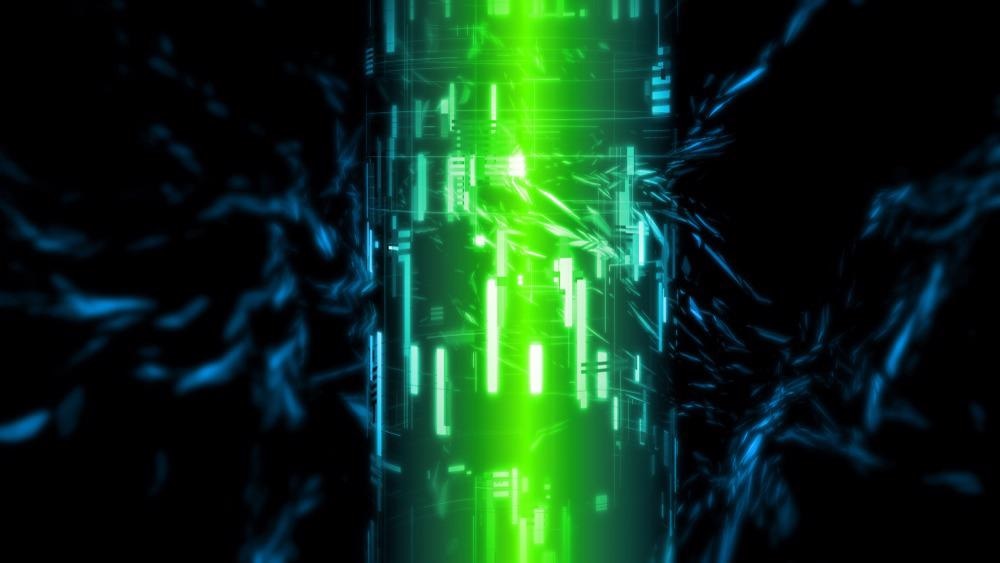
Study: Storage of Garnet Solid Electrolytes: Insights into Air Stability and Surface Chemistry. Image Credit: kkssr/Shutterstock.com
Background
Solid-state lithium metal batteries (SSLMBs) are made possible by the development of fast lithium-ion conductors, which are expected to enable next-generation high-energy-density batteries and eliminate the safety issues related to the liquid lithium-ion batteries. Oxide solid electrolytes (OSEs) stand out among the many solid electrolytes (SEs) because of their robust mechanical properties, ease of manufacture, and thermal and chemical stability.
Because of its strong ionic conductivity and better electrochemical/chemical stability of a Li metal anode, the Li-rich garnet-type Li7La3Zr2O12 (LLZO) is one of the most promising OSEs. Material preparation, cation replacements and dopants, ion transport mechanisms, ion conductivity, and interface engineering have all made significant progress in the last decade for LLZO.
A detailed understanding of the air passivation layer's (APL) response mechanism and impedance characteristics may aid in the development of techniques to enhance the quality of the SE/Li interface and simplify cell assembly. Unfortunately, for APL analysis, general characterization techniques are impractical.
About the Study
In this study, the authors discussed the air stability qualities of Li6.4La3Zr1.4Ta0.6O12 (LLZTO), such as the genesis of instability, parameters of the APL, and the air passivation mechanism. The first effort focused on the development of a systematic methodology for the investigation of the air stability-based characteristics of LLZTO, such as the properties of the APL such as influencing factor, thickness, microstructure, component, and impedance.
The origin of the air passivation mechanism and instability were also proposed. The second goal was to assess the influence of the APL on interfacial resistance, research the APL's thermostability, and improve an annealing technique to mitigate the APL's detrimental effect at the "Li/LLZTO" interface.
The team investigated the air instability by using freshly dissolved LLZTO ceramic cross-sections that were not polished. The polishing technique for fragile ceramics resulted in a mechanical damage layer forming on the surface, which caused significant chemical and morphological alterations. The storage characteristics of three distinct LLZTO morphologies (i.e., ceramic cross-section, powders, and pellet) were studied over a long-time scale. The recovery effect of thermal treatment at various temperatures, as well as the related electrical properties were also demonstrated.
More from AZoM: Which Alloys Are Prone to Corrosion, and How Can We Prevent This?
The researchers used the 15-level X-ray photoelectron spectroscopy (XPS) depth profiling to analyze the chemical binding and elemental content progression in the APL on freshly dissolved LLZTO cross-sections. As a function of the sputtering depth, this characterization was used to show a continuous evolution of the above-mentioned features. The influence of APL on the interfacial resistance was assessed, the APL's thermostability was explored, and further, an annealing technique was refined to mitigate the APL's detrimental effect at the "Li/LLZTO" interface.
Observations
The primary pollutants in the APL, according to 15-level X-ray photoelectron spectroscopy depth profiling and thermogravimetric analysis, were Li2CO3, LiOHxH2O, and Li6.4xHxLa3Zr1.4Ta0.6O12, and the concentrations increased with exposure duration. The LiOHxH2O formed by the Li+/H+ exchange reaction was a crucial step in the production of APL. They resolved completely at 700 °C, with just a little content of the Li-poor phase, i.e., La2Zr2O3 formed. The surface morphology and characteristics could be recovered when the surficial APL-contaminated layer was efficiently removed by using a heat treatment procedure at 700 °C for 3 hours.
The Li/LLZTO interface impedance dropped to 3 from 200 Ω, and the critical current density increased from 0.2 to 0.65 mA cm-2. At a current density of 0.1 mA cm-2, the symmetric Li/LLZTO/Li cells worked for over 4000 hours. For more than 1000 hours at a larger current density of 0.2 mA cm-2, no noticeable short-circuit signal was recorded.
Conclusions
In conclusion, this study elucidated that a practical heat treatment at 700 °C for 3 h is a viable and simple way of removing the surficial APL entirely. The authors believe that the findings of this study add to our knowledge of LLZTO's air stability and pave the way for garnet-based SSLMBs to be used in real applications. Furthermore, they mentioned that the proposed systematic methodology may spark new research into air stability-related difficulties for a broader range of solid electrolytes.
It was observed that the ameliorative interfacial contact is responsible for the considerable improvement in Li/LLZTO/Li electrochemical performance. The results contributed to a better understanding of LLZTO's air stability and provided a systematic description of the produced contaminated layer. The team emphasized that this technology could lead to new research into air stability difficulties in larger solid-liquid electrolyte systems and the proposed heat treatment approach could be used as a crucial pre-treatment for better surface modification on LLZTO, paving the way for all-solid-state battery applications in the future.
Disclaimer: The views expressed here are those of the author expressed in their private capacity and do not necessarily represent the views of AZoM.com Limited T/A AZoNetwork the owner and operator of this website. This disclaimer forms part of the Terms and conditions of use of this website.
Source:
Jia, P., Ji, X., Zheng, P., et al. Storage of Garnet Solid Electrolytes: Insights into Air Stability and Surface Chemistry. ACS Applied Energy Materials (2022). https://pubs.acs.org/doi/10.1021/acsaem.2c00452