By Surbhi JainReviewed by Susha Cheriyedath, M.Sc.May 18 2022
In an article recently published in the journal Additive Manufacturing Letters, researchers presented an elastic-plastic fracture mechanical approach to assessing uniform fatigue damage tolerance for additively manufactured and cast Al-Si alloys.

Study: Uniform Fatigue Damage Tolerance Assessment for Additively Manufactured and Cast Al-Si Alloys: An Elastic-Plastic Fracture Mechanical Approach. Image Credit: Alex_Traksel/Shutterstock.com
Background
Additive manufacturing (AM) and casting allow for the exact creation of complicated external and internal geometries and stiffnesses tailored to the local load flow. Due to their excellent castability and low density, aluminum-silicon (Al-Si) alloys are widely employed in the automobile and railway industries.
Despite the fact that AM and casting methods have improved in recent decades, process-induced faults can only be mitigated, not totally prevented. As a result, process-induced faults must be taken into account appropriately for the safe design of highly loaded parts.
Murakami's technique and the usual modification for light metal alloys according to the Murakami-Noguchi approach can be used to describe the effect of flaws on fatigue strength.
(a) Binary Al-Si phase diagram and (a) representative micrograph of hypoeutectic Al-Si alloy containing dendritic α-Al solid solution (light areas) and interdendritic Al-Si eutectic (dark areas). Image Credit: Tenkamp, J. et al., Additive Manufacturing Letters
About the Study
In this study, the authors discussed the development of a uniform damage tolerance evaluation based on Murakami and Shiozawa's fracture mechanical techniques for a reliable defect-based mechanical design of fatigue-loaded structures.
Murakami and Shiozawa's linear-elastic fracture mechanical (LEFM) approaches were used to calculate defect-based lifetime curves, in which the cyclic stress intensity factor (ΔK) at the failure-initiating defect (√area) was used to describe local stress concentration conditions, which resulted in K-N curves rather than nominal stress-based S-N curves.
The researchers employed the cyclic stress-strain (CSS) behavior for a plasticity modification of the LEFM technique by calculating the effective cyclic J integral (Δ Jeff) to draw J-based K-N curves called Kj-N curves.
For additively made and cast Al-Si materials, the effect of the process-induced microstructure, flaws, porosity, and stress-strain behavior on fatigue and fatigue damage-tolerant (FDT) behavior was investigated.
Power bed fusion with a laser beam (PBF/LB) was used to process the AlSi10Mg material, while sand-casting (SC) and die-casting (DC) were used to manufacture the cast AlSi7Mg materials. The AM material was evaluated as-built, whereas the cast materials were adjusted in terms of porosity, eutectic Si precipitates, dendritic arm spacing, and cooling rate. Tensile and incremental step tests were used to determine the quasi-static (QSS) and CSS characteristics, respectively.
The team investigated the impact of AM and casting method on defect distribution, microstructure, fatigue behavior, and CSS for process-specific standard Al-Si alloys AlSi10Mg and AlSi7Mg. To determine their limitations, the use of contemporary methodologies for damage tolerance assessment was reviewed.
Furthermore, the findings were coupled to novel elastic-plastic fracture mechanical techniques in order to overcome these limitations and allow for a unified fatigue damage tolerance assessment of AM and cast Al-Si alloys. The fatigue behavior was described using both traditional and new methodologies, including Murakami-relativized Noguchi's S-N curves and Woehler's S-N curves based on estimated hardness- and defect-based fatigue limits.
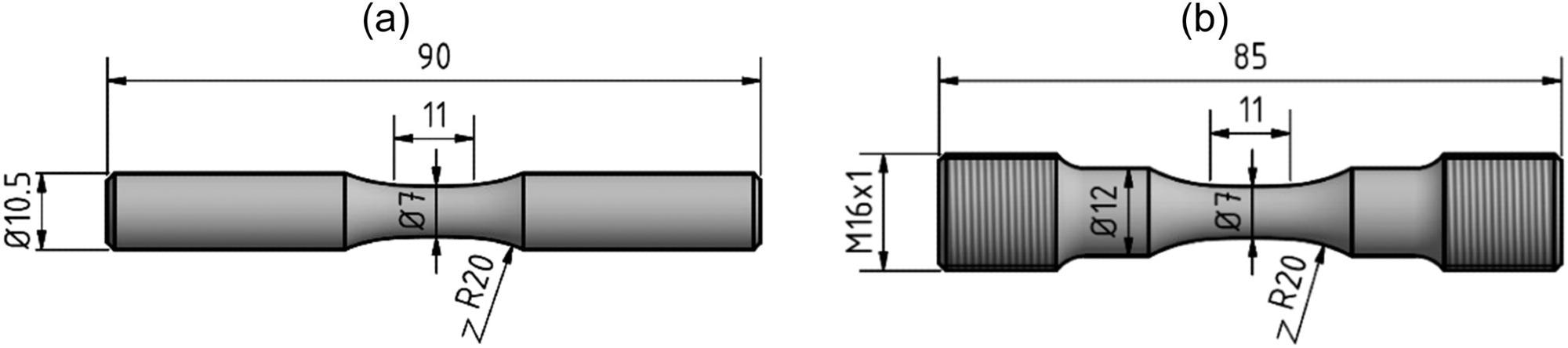
Geometries: (a) Strain-controlled tensile and LCF tests; (b) stress-controlled HCF tests. Image Credit: Tenkamp, J. et al., Additive Manufacturing Letters
Observations
For compression-tension loading, the fatigue limit of AM alloy was dramatically reduced, especially when the ratio of compressive loading (stress ratio, R) was raised to -2. Furthermore, for R = -1, stress sensitivity increased as defect size decreased.
When R was equal to 0.1, the FDT was found to be much lower as compared to when R was equal to -1. AM had a quasi-static 0.2% yield strength (YS) that was comparable to the selected SC and DC, but the 0.02% YS was lower, and the ultimate tensile strength was much higher. The YS of SC1 eventually surpassed that of DC1 by 5%. The strain hardening coefficients of DC1p2-QSS curves were lowered by as much as 10%. For DC1p2 and DC1, the cyclic strain hardening coefficient increased by 22% and 7%, respectively.
The fatigue behavior could not be described in terms of a unified fatigue design of AM and casting material by using LEFM-based K-N curves. The EPFM approach allowed for a unified FDT assessment of AM and cast Al-Si alloys for the HCF regime.
AM materials had higher quasi-static strength but lower cyclic strength than cast materials, whereas cast materials had higher cyclic strength due to the significant cyclic hardening characteristic. Murakami-Noguchi was able to correctly anticipate the fatigue limit of cast materials, but the fatigue limit of AM materials was greatly overestimated.
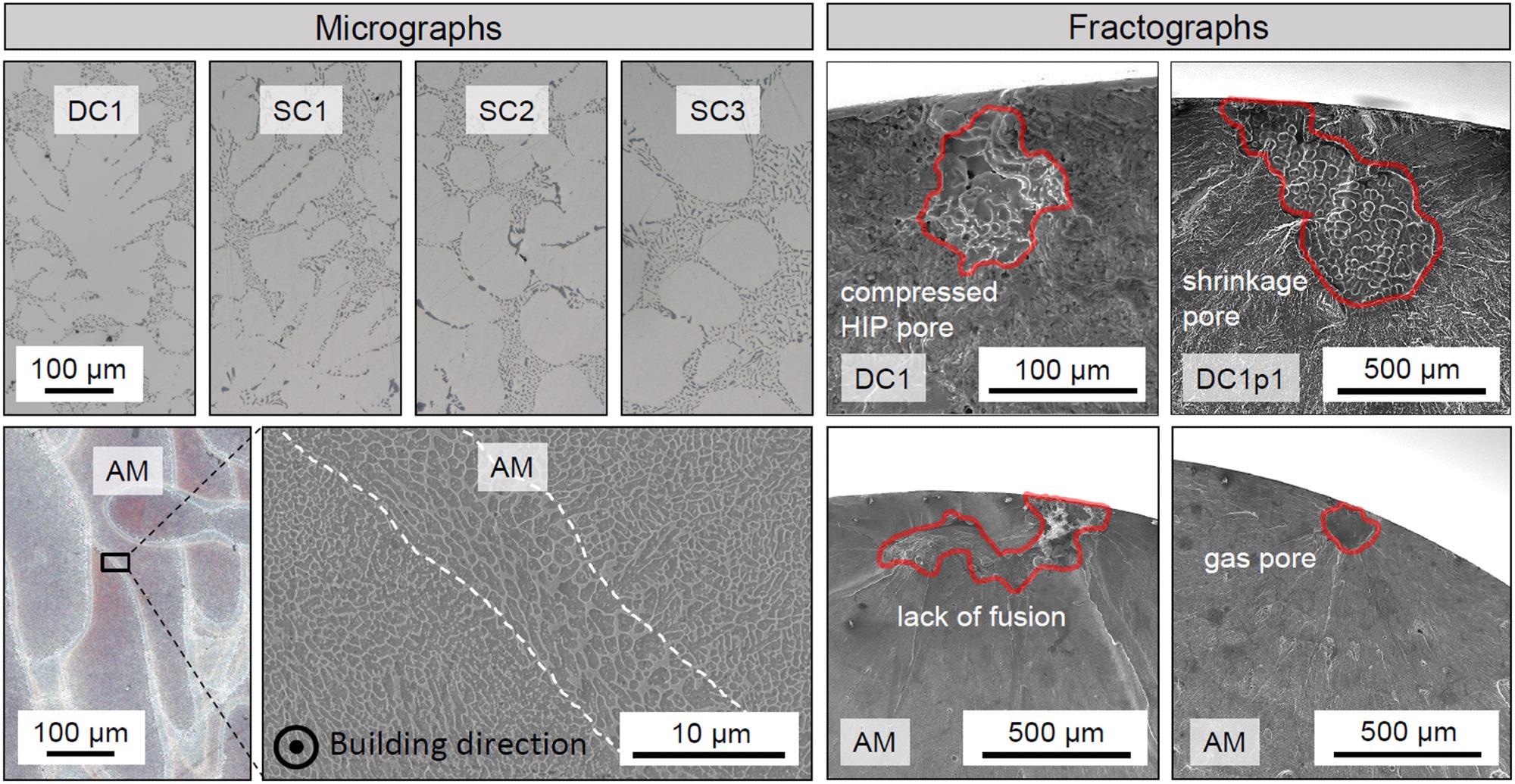
Representative OM and SEM micrographs (left) as well as SEM fractographs (right) of DC and SC AlSi7Mg alloy (top) and AM AlSi10Mg alloy (bottom). Image Credit: Tenkamp, J. et al., Additive Manufacturing Letters
Conclusions
In conclusion, this study elucidated the translation of the J integral to the stress intensity factor (SIF) dimension, known as J-SIF or Kj, which was employed in the J-based Shiozawa diagram using Kj-N curves. This change allowed for a consistent and accurate FDT evaluation regardless of process-specific microstructure, plasticity, or porosity characteristics. As a result, a standard FDT legislation for the HCF regime and the FDT limit or fatigue limit could be discovered.
The authors mentioned that using the cyclic stress-strain behavior, specific defect distribution, and stress ratio, the uniform FDT limit, and the uniform FDT law may be utilized to construct Haigh diagrams and synthetic S-N curves for Al-Si alloys.
Disclaimer: The views expressed here are those of the author expressed in their private capacity and do not necessarily represent the views of AZoM.com Limited T/A AZoNetwork the owner and operator of this website. This disclaimer forms part of the Terms and conditions of use of this website.
Source:
Tenkamp, J., Stern, F., Walther, F., Uniform Fatigue Damage Tolerance Assessment for Additively Manufactured and Cast Al-Si Alloys: An Elastic-Plastic Fracture Mechanical Approach. Additive Manufacturing Letters 100054 (2022). https://www.sciencedirect.com/science/article/pii/S2772369022000287