A team of researchers recently published a paper in the journal Advanced Science that reviewed the use of elastic fabrics/fibers for bioelectronics and wearables.
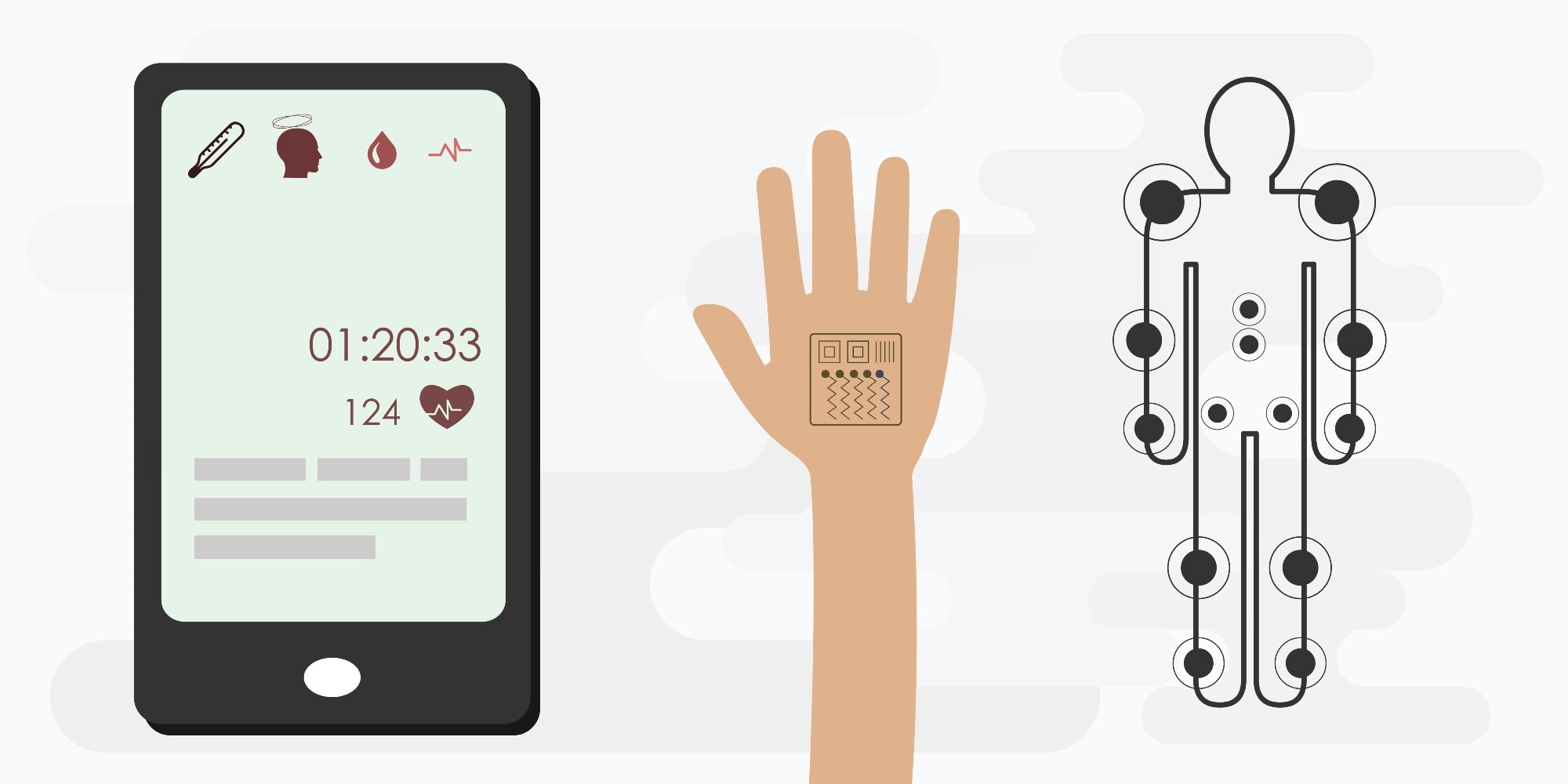
Study: Elastic Fibers/Fabrics for Wearables and Bioelectronics. Image Credit: magic pictures/Shutterstock.com
Background
Bioelectronics and wearables depend on breathable interface devices with smart functionality, biocompatibility, and bio-affinity for interactions between objects, organisms, and their surrounding environment.
Elastic fabrics/fibers possessing mechanical adaptivity to different complex substrates and deformations are considered suitable as scaffolds, dressings, substrates, carriers, and fillers during the fabrication of bio-interfaces for plants, organs, skin, and the human body to realize several functions.
These functions include intervention therapy, disease diagnosis, health monitoring, augmented virtuality, perception, sensing, and energy exchange. In this study, the authors reviewed the use of elastic fabrics/fibers for bioelectronics and wearables. They also identified the advantages and challenges of different elastic fiber/fabric and yarn fabrication methods.
Advantages and Challenges of Different Elastic Fiber, Fabric, and Yarn Fabrication Methods
Fiber
Fibers with complex geometries, such as an island, core-shell, and co-spinning, can be fabricated using the wet spinning method. Single-step fabrication and the use of different raw materials are the other advantages of this method. However, process discontinuity, slow processing, and inferior uniformity are the major drawbacks of the wet spinning method.
Diversity of the prefabs, accurate integration, and component tunability are the major advantages of the thermal drawing method. However, the high cost of equipment and restrictions on the use of input materials are the significant challenges of using this method.
Low cost, simple operation, and integration diversity are the key benefits of the finishing method. Fibers prepared using this method possess diverse functionality and are used in batteries and sensors. Adverse effects on fiber properties, inferior durability, and poor structure uniformity are the major disadvantages of the finishing method.
Yarn
Yarn with structural diversity and elasticity can be mass-produced using the twisting method. However, poor performance and limitations in device fabrication are the significant challenges of this method.
The finishing method is used to fabricate yarn with diverse functionality in a facile and inexpensive manner. An extensive range of input materials can be used in this method. Adverse impact on the yarn twisting effect, inferior durability, and lack of yarn wearable merits are the major drawbacks of this method.
Fabric Textile
High-performance fabric textiles with good mechanical strength and customized device patterns can be fabricated using the weaving method. However, achieving precise fabrication of contact junctions for various devices, low structural elasticity, and limitations of the raw materials in providing electrical and mechanical stability to the final product are the major disadvantages of this method.
Inherent structural elasticity, high mechanical strength, easy incorporation of e-yarn, and scalability are the major advantages of using the knitting method for fabric textile fabrication. However, knitting has similar drawbacks to the weaving method.
Large-scale production, diversity in fabrics, and incorporation of different components are the crucial benefits of using the nonwoven method for fabric textile fabrication. However, complexities in the fabrication process and poor mechanical properties of the fabric textiles are the significant challenges of this method.
High throughput, precise patterning, and versatility in the fabrication process are the major advantages of the printing method. However, limitations on the use of new materials, expensive equipment, and complex process parameters are the major drawbacks of this method.
An extensive range of raw materials can be used to fabricate fabric textiles with large-scale functionality using the finishing method. However, low fabrication accuracy, deterioration of textile wearability, and inferior durability and washability are the key disadvantages of this method.
Elastic Fibers/Fabrics for Wearables and Bioelectronics
Fiber fabricated using the wet spinning method is typically used in batteries, triboelectric nanogenerators (TENGs), and sensors and for drug delivery and biosensing. Fibers prepared thermal drawing method can be used to fabricate fiber for batteries, sensors, TENG, and thermoelectric nanogenerators (TEGs).
Yarn fabricated using the twisting and finishing methods is used in TENG, piezoelectric nanogenerator (PENG), supercapacitors (SCs), TEG, TENG, and sensors, and for thermal management. Fabric textile prepared using the weaving/knitting/nonwoven/printing/finishing method is used in sensors, TEG, battery, PENG, TENG, and SCs, and for personal protection and thermal management.
Fabric-based PENG three-dimensional (3D)-printed using poly(vinylidene fluoride-trifluoro ethylene) (P(VDF-TrFE)) and barium titanate demonstrated a maximum power density of 1.4 μW cm−2 and 300% stretchability.
Similarly, fabric-based TENG fabricated using the electrospinning/electrospraying/screen printing method and poly(vinylidene fluoride-co-hexafluoropropylene) (PVDF-HFP), poly(styrene-b-(ethylene-co-butylene)-b-styrene) (SEBS), liquid metal, and silver flakes as raw materials displayed a maximum power density of 219.66 mW m−2 and can withstand 490% strain.
Yarn-based TEG fabricated using the coating method and poly(3,4-ethylenedioxythiophene) polystyrene sulfonate (PEDOT:PSS) and Lycra as raw materials can withstand almost 1000% strain.
Styrene-isoprene-polyaniline (SIS-PANI) elastic sensing membrane based on SIS nanofibers can detect complex motion behaviors, such as twisting and bending, between 1°–180° angles and 3–18 rpm deformation speeds. Moreover, the 300–10,000 nm thick strain sensor can precisely determine various mechanical stimuli continuously.
An ultrathin breathable electronic skin (e-skin) with non-inflammation and high stretchability was prepared by depositing a gold layer on an electrospinning polyvinyl alcohol (PVA) nanofilm, which is eventually dissolved in situ on the skin.
The device demonstrated high adhesion to the skin and exceptional resistance stability after 10000 finger bending cycles with 40% elongation, indicating the excellent electrical and mechanical reliability of elastic on-skin conductors.
A nanoporous silver film was coated on polydopamine (PDA)-modified nano polyethylene (nanoPE) to fabricate a textile with tunable heat transmission properties. The nano silver/PE demonstrated low infrared radiation emissivity of 10.1%.
MXene/PDA/nickel (II) ion-coated spandex yarn was prepared using the dip-coating method as antibacterial textile. The yarn-based strain sensor effectively sterilized bacteria upon near-infrared radiation.
Similarly, PVA-co-PE electrospinning nanomembranes were modified using the dip-coating method to fabricate rechargeable antiviral and antibacterial nanofibrous membranes.
The output electricity generated by a nanogenerator composed of copper/polytetrafluoroethylene (PTFE) layer as electronegative material and copper layer as electropositive material on both sides of a polyethylene terephthalate (PET) film accelerated the closure of a cutaneous wound in a mouse within three days compared to the 12 days required in a normal healing process.
Chitosan–reduced graphene oxide sensing films fabricated using the spray method was used to develop a self-powered wearable acetone sensor for real-time diabetes diagnosis and respiratory monitoring. The film demonstrated a 27.89% sensing response at 10 ppm acetone concentration, which is significantly higher compared to the response shown by pure chitosan film.
Functionalized cotton fibers synthesized by coating alginate/poly(ethylene glycol) diacrylate (PEGDA) hydrogels, carbon ink, and drug-loaded heat-sensitive poly( N-isopropyl acrylamide) (NIPAM) particles for wound healing displayed their effectiveness in controllable transdermal drug release.
Similarly, a superparamagnetic graphene oxide (SPGO) nanofiber film-based implantable anticancer device prepared for breast cancer treatment effectively enabled wound reconstruction, noninvasive detection, and drug release.
Conclusion
Significant progress has been made in improving the sensitivity, stretchability, electrical output, power density, and bio-friendliness of electronic textiles for applications in bioelectronics and wearables. For instance, superelastic fiber/fabric sensors were developed to monitor complex movements in real-time, while fiber/fabric-based TENG was developed for energy storage, utilization, and conversion to ensure long-term operation.
However, the existing development methods and products do not meet the requirements of practical applications in terms of diverse environment adaptivity, signal fidelity and sensitivity, high electrical output, and industrial-scale manufacturing. Thus, more research is required to eliminate the technology and material limitations to achieve effective integration of device components and good interface adaptivity between components and the substrate and device for continuous device operation with high fidelity, sensitivity, and stability.
More from AZoM: The Current State of the Global Semiconductor Market
Disclaimer: The views expressed here are those of the author expressed in their private capacity and do not necessarily represent the views of AZoM.com Limited T/A AZoNetwork the owner and operator of this website. This disclaimer forms part of the Terms and conditions of use of this website.
Source:
Zhang, Y., Zhou, J., Zhang, D., et al. (2022) Elastic Fibers/Fabrics for Wearables and Bioelectronics. Advanced Science. https://onlinelibrary.wiley.com/doi/10.1002/advs.202203808