Quantifying the impact of temperature on powder flow and bulk properties for more effective process optimization.
Powders are handled at elevated temperatures across a range of industrial applications. For example, catalysts typically operate at elevated temperatures to achieve maximum productivity, while additive manufacturing (AM) build chambers are heated to reduce warpage and thermally induced stress in the finished component.
Pasteurization and dehydration are good examples of heat treatment processes applied to extend the shelf-life of products by reducing microbial populations or preventing fungal growth, particularly within the food industry. The pharmaceutical sector relies on melt granulation and hot-melt extrusion to achieve target bioavailability and controlled release rates in solid dosage forms.
Over recent years the importance of characterizing powders under process relevant conditions has become increasingly well-established, but most testing is still carried out at ambient temperature. As a consequence, powders may exhibit unpredictable performance in many unit operations, leading to issues such as stoppages, downtime, and out-of-specification products.
At elevated temperature solid particles may deform elastically or plastically, moisture will migrate, and the density of entrained gas will reduce. These and other changes directly impact bulk powder properties such as flowability, compressibility, and permeability that define process and product performance.
In the case studies below, we consider how temperature affects powder behavior and the requirements for elevated temperature testing. Experimental data is presented that quantifies the extent to which temperature changes the flowability of two powders – a pharmaceutical excipient and a polymer powder for AM. The results highlight the difficulty of predicting such changes and the need for appropriate testing.
Understanding Powders
Bulk powder behavior is governed by interactions between their constituent elements. These include solid particles, any liquid present on the surface of or within particles, and gas, typically entrained air (see figure 1). Even at ambient temperature, these interactions are numerous and complex. A significant number of particle properties influence powder behavior including:
- Particle size and distribution
- Shape
- Surface area and texture
- Density
- Porosity
- Potential for electrostatic charge
However, system variables, such as the degree of aeration or consolidation or the amount of moisture present, are also highly influential. The transformation in flowability that can occur as a result of deaeration or the ingress of moisture will be familiar to many processors.
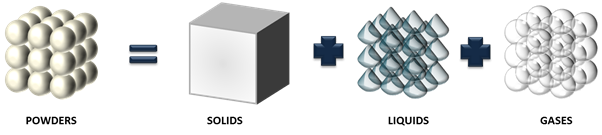
Figure 1. Bulk powders consist of solid particles, liquid, and entrained gas. Image Credit: Freeman Technology
This underlying complexity explains the difficulty of mathematically modeling powder behavior and industrial reliance on powder testing. Over recent years there has been an increasing need to measure bulk properties, such as flowability, under conditions that simulate the application or process of interest. However, test equipment has historically presented little opportunity to investigate the impact of temperature.
Particle Properties - Freeman Technology
Video Credit: Freeman Technology
The Impact of Elevated Temperature
Increasing temperature above ambient conditions can have multiple effects on a powder with all three constituents potentially affected.
Amorphous solids, principally polymers, exhibit a glass transition temperature (Tg), a temperature at which the material changes from a more brittle, glass-like state to one that is softer and more ductile. This transition can occur at relatively low temperatures. For example, the Tg of many polyamides, a type of polymer commonly used for 3D printing, is typically in the region of 30 – 50 oC1. Particles heated beyond their Tg may exhibit plastic deformation and a permanent change in particle morphology and in surface properties such as texture and area. Inter-particular cohesive interactions may also change, and particles can begin to agglomerate, forming larger clusters with significantly different characteristics. Melting of the fat content of a particle can similarly impact bulk powder properties and may also cause the adhesion of particles, for example to the surface of processing equipment.
Any liquid in a powder becomes more prone to evaporation as temperature increases. There is a tendency to associate low moisture levels with improved powder flowability, but this is an unreliable assumption2. Loss of liquid undoubtedly has the potential to disrupt any capillary bonding in a powder, thereby allowing particles to move more independently relative to one another. However, in certain powders water lubricates particle-particle interactions while in others it helps to dissipate accumulated electrostatic charge; both effects may improve flowability. Counter-intuitively, loss of water may therefore be associated with a deterioration in flow behavior in certain instances.
Finally, increasing temperature increases the pressure of any entrained gas and reduces its density, depending on the conditions under which the powder is held. Moisture levels in the gas, as opposed to the particles, may also decrease at elevated temperatures.
The net impact of these changes is unpredictable, for the same reasons that powder behavior cannot be predicted from a fundamental knowledge of particle and process variables at ambient temperature. Assessing the effect of temperature calls for testing at a suitably elevated temperature.
Requirements for Elevated Temperature Testing
It is useful to review industrial applications where elevated temperature measurements are relevant. While many processes are intentionally operated at elevated temperature, powders may also be inadvertently subject to handling or processing at above ambient temperature when:
- Stored in an area or facility without environmental control.
Diurnal temperature variation is a function of geography, but changes can be as high as 30 oC. In an uncontrolled storage environment, such changes may also be magnified by accompanying variability in relative humidity.
- Heat is generated by friction in processes such as milling or blending.
For example, processing in a hammer mill can increase the temperature of a powder from ambient to as much as 90 oC 3. Certain types of mill are associated with much lower temperature increases, for example, jet mills, and designs may be modified for heat dissipation, to handle heat-sensitive materials such as pharmaceutical actives. Understanding how the physical characteristics of a powder may be changed by a relatively modest increase in temperature is useful, complementary information when it comes to assessing requirements for such designs.
- Intermediate materials of different temperatures are blended.
Blending is a good example of a process where performance has been shown to correlate directly with flowability4. In a system where hot powders are blended with cooler ones, for example with fresh material or additives, any resulting incremental temperature rise may directly impact flowability and by extension blending behavior.
These factors make somewhat uncontrolled rises in temperature common across the powder processing industries. However, there are many processes that directly call for powder handling at elevated temperatures. In the following sections, we take a closer look at examples from the pharmaceutical and AM industries.
Case Study 1: Investigating the Behavior of Pharmaceutical Excipients at Elevated Temperature
In an experimental study, the flow properties of two common lactose excipients – GranuLac 70 and GranuLac 140 (Meggle Wasserburg GmbH & Co, KG) were measured at elevated temperature. Particle size data for the two samples are shown in Table 1.
Table 1. Particle size distribution data for GranuLac 70 and GranuLac 140. Source: Freeman Technology
Lactose Excipient |
Particle Size Distribution, µm |
D10 |
D50 |
D90 |
GranuLac 70 |
16 |
107 |
213 |
GranuLac 140 |
8 |
46 |
127 |
In the first experiment, the flowability of both excipients was measured at temperatures from 25 – 100 oC, holding the sample at each setpoint temperature for 30 minutes prior to measurement. In a further study, flowability was quantified as a function of storage time at 80 oC, for periods of up to 4 hours. All tests were carried out using the FT4 Powder Rheometer® (Freeman Technology, Tewkesbury, UK) which quantifies flowability via dynamic measurements, generating metrics such as Flow Energy (as reported).
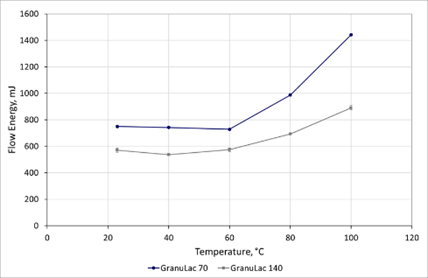
Figure 2. Measurements of Flow Energy as a function of temperature show that the flowability of the lactose excipients is substantially changed by increasing temperature. Image Credit: Freeman Technology
The results demonstrate the high repeatability of the measurements and show clearly that the Flow Energy of both excipients remains essentially unchanged up to a temperature of 60 oC. A marginal decrease in Flow Energy is observed for the GranuLac 140 at 40 oC which may be attributable to a reduction in capillary bonding. At temperatures in excess of 60 oC Flow Energy increases significantly, exhibiting a non-linear, repeatable relationship with a temperature that is different for each excipient. GranuLac 70 is more sensitive to temperature, with a Flow Energy at 100 oC around 90% higher than at ambient temperature.
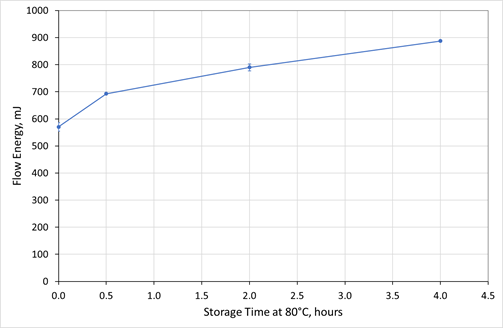
Figure 3. The Flow Energy of GranuLac 140 increases steadily with storage time when the excipient is stored at elevated temperature (80 oC). Image Credit: Freeman Technology
Figure 3 shows how the Flow Energy of the GranuLac 140 increases as a result of storage for prolonged periods at a temperature of 80 oC. Again, a repeatable, non-linear relationship is observed, culminating in a substantial rise in flow energy, within a relatively modest timeframe.
These simple studies show that at industrially relevant temperatures the flowability of these lactose excipients changes significantly, and in an unpredictable way. Flow Energy values have been robustly correlated with performance in a number of processes, so these results suggest that the behavior of these powders in industrial unit operations will be altered by increasing temperature. Notably, the sensitivity to temperature of the two materials is substantially different despite their similar chemistry. This suggests that sensitivity to temperature is likely associated with physical properties such as particle size, morphology, and the strength of inter-particular interactions.
Case Study 2: Rationalising the Performance of Polymeric AM Powders via Elevated Temperature Powder Testing
Two polymer powders with comparable flow properties at ambient temperature were found to perform differently in an AM printer. Polymer A gave acceptable printing performance as a virgin powder, and when recycled, while Polymer B was associated with poor part quality, especially when recycled. Samples of each powder were subject to extensive flowability and permeability testing at ambient temperature under a range of stress regimes. Flowability measurements were then carried out at elevated temperature up to the printing temperature, 113 oC. All tests were carried out using an FT4 Powder Rheometer (Freeman Technology, Tewkesbury, UK).

Figure 4. Flowability values under moderate (a) and low stress (b) conditions along with permeability measurements (c), all carried out at an ambient temperature, indicate similarity between the two powders. Image Credit: Freeman Technology
Data measured at ambient temperature (see figure 4) suggests that the two powders are similar. (Basic) Flow Energy and Specific Energy values, which quantify flowability in a moderate and low-stress state, under confined and unconfined test conditions5, show little difference and permeability data are also similar. Shear stress analysis and aerated flow measurements provide further evidence of the similarity between the samples (data not shown). However, flowability measurements at elevated temperature, at temperatures across the range 25 to 113 oC do differentiate the samples (see figure 5).
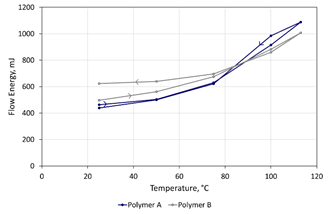
Figure 5. Elevated temperature testing differentiates the polymer feedstocks with Polymer B exhibiting a more modest change in Flow Energy as the temperature is increased, but greater hysteresis upon cooling. Image Credit: Freeman Technology
Both feedstocks are sensitive to temperature, with Flow Energy exhibiting a non-linear, repeatable relationship with temperature. However, Polymer A was found to be more sensitive than Polymer B, with flow energy increasing by >120% c.f. 90% as a result of increasing temperature from 25 to 113 oC. Upon cooling, the behavior of the powders was further differentiated. The Flow Energy of Polymer A returned to a similar level to that observed prior to heating, while Polymer B displayed significant hysteresis, indicating a permanent change in flow properties after heating. The ambient flow energy of Polymer B was around 25% higher post-heating.
These results illustrate the potential for elevated temperature testing to detect differences that are indiscernible at ambient temperature. Flow Energy values have been directly correlated with printing performance in the previous studies5 so materials that generate different values, under relevant test conditions, would be expected to perform differently in AM processes.
In Conclusion
Testing powders under process-relevant conditions has become an established good practice with processors increasingly recognizing the value of being able to characterize powders under different stress and strain regime conditions. Technology for testing at elevated temperature further improves the match between powder testing capabilities and industrial requirements. The results presented here show how elevated temperature measurements of flowability can be used to more robustly assess and differentiate the behavior of powders at temperatures routinely encountered in industrial applications. Such testing presents new opportunities to build a better understanding of powder performance and optimize vital processes.
References and Further Reading
- ‘Glass transition temperature’ Omnexus, the materials selection platform. Available to view at: https://omnexus.specialchem.com/polymer-properties/properties/glass-transition-temperature
- T. Freeman et al. ‘Controlling the impact of humidity’ Process Industry Informer June/July 2012 (Solids Handling Supplement).
- ‘Ask an expert (F. Surville)’ Tablets and Capsules, Solid Dose Digest Available to view at: https://www.tabletscapsules.com/enews_tc/2018/issues/tcnews_04_23_18expert.html
- ‘Using powder characterisation methods to assess blending performance’ Whitepaper available to download at: https://www.freemantech.co.uk/news/using-powder-characterisation-methods-to-assess-blending-behaviour
- J. Clayton and O.Ghita ‘Pushing for performance in polymer powders’ Nov 2019. Available to view at: https://www.tctmagazine.com/blogs/guest-column/pushing-for-performance-in-polymer-powders/

This information has been sourced, reviewed and adapted from materials provided by Freeman Technology.
For more information on this source, please visit Freeman Technology.