A metamaterial is any material that was engineered so that a property not found in naturally occurring materials could be exploited. Designed right, metamaterials can affect sound waves, electromagnetic radiation, light, and even earthquakes in ways that bulk materials cannot. This article explores the different types of metamaterials, their history, uses, and modern applications.
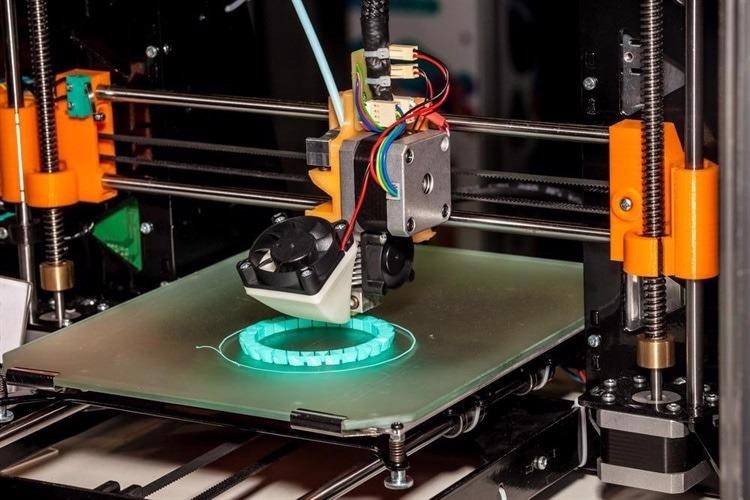
Image Credit: Alexander Tolstykh/Shutterstock.com
To achieve their unique properties, metamaterials are designed in repeating patterns at scales that are smaller than the wavelengths of the energy they affect. Their properties do not come from aspects of their base materials, but from their uniquely engineered structure.
The shape, geometry, size, orientation, and arrangement of surface structures on metamaterials are what give them their unique properties. Carefully designed, these physical features enable metamaterials to manipulate electromagnetic waves, for example by blocking, absorbing, enhancing, or bending them.
The main focus of research into metamaterials has been centered on materials whose index of refraction is negative for specific wavelengths. These are referred to as negative-index metamaterials.
The potential applications for metamaterials are diverse and promising. They have been proposed as candidates for optical filtering, medical devices, remote aerospace operations, sensor detectors, solar power management, crowd control, radomes, antenna lenses, and even earthquake protection.
Lenses made of metamaterials may even enable imaging below the diffraction limit that prevents conventional optical lenses from magnifying any further. Gradient-index metamaterials can even provide a kind of “invisibility” based on these optical applications.
Further Reading: Developing a Highly Configurable Metamaterial with 3D Printing
Research into metamaterials is interdisciplinary by nature. Electrical engineers, electromagnetic researchers, classical optics scientists, solid-state physicists, microwave engineers, antenna engineers, optoelectronics developers, materials scientists, nanotechnology researchers, and semiconductor engineers all work together on the development of metamaterials around the world.
Engineering Metamaterials for Different Wavelengths
Metamaterials can be classified according to the different wavelengths they are engineered to operate on. Different wavelength sizes correspond to different types of energy, but the functioning principle of metamaterials is the same.
Electromagnetic Metamaterials
Electromagnetic metamaterials affect electromagnetic waves that interact with their surface features, which are smaller than the wavelength of electromagnetic radiation.
The widest wavelength of electromagnetic radiation are microwaves, and metamaterials designed to work with these have features up to millimeters apart. Arrays of electrically conductive elements (loops of wire, for example) with suitable inductive and capacitive characteristics can be made to manipulate microwaves.
For photonic radiation, metamaterials’ structure must be ordered on the scale of nanometers. Frequency-selective surfaces like diffraction gratings, optical coatings, and dielectric mirrors, as well as photonic crystals, show similarities to photonic metamaterials. They are usually considered distinct from classic metamaterials, however, due to their heterogeneity.
Plasmonic metamaterials have been developed that use surface plasmons, parcels of electrical charge that oscillate together at the surface of metals.
Electromagnetic metamaterials are the main type to be studied and developed, as they have the most applications identified currently.
Elastic Metamaterials
Elastic metamaterials create a negative index of refraction in non-electromagnetic materials by using different parameters. Elastic metamaterials can behave either as liquids or solids over their limited range of frequencies, and may enable new applications in sound and seismic energy management.
Acoustic Metamaterials
Just like electromagnetic waves, sonic waves can also exhibit negative refraction. As such, acoustic metamaterials have been developed to control, direct, and manipulate sound’s passage through gas, liquid, or solid materials.
Analogous to permittivity and permeability in electromagnetic metamaterials, bulk modulus, and mass density can be manipulated to control sound waves. This enables metamaterials to form a resonant system that is excited by the correct sonic frequencies.
extreme mechanical metamaterials
Video Credit: Inside Science/Shutterstock.com
Structural Metamaterials
Projection micro-stereolithography is used to create micro-lattices like trusses and girders to manufacture structural metamaterials. This technique has led to new materials that are four times as stiff as conventional aerogel, with the same low density. They can withstand loads from 160,000 times their weight and even higher.
Nonlinear Metamaterials
Nonlinear media can be used in the fabrication of metamaterials to create materials whose properties change as incident waves change direction. Nonlinear media like these are crucial in the development of nonlinear optics.
If the metamaterial effective dielectric permittivity of a medium is small enough (known as epsilon-near-zero media), they can exhibit remarkable nonlinear optical properties. Tweaks to the materials’ negative refractive index can also be used to tailor phase-matching conditions for nonlinear optical structures.
Metamaterials’ Applications
While industrial techniques have not yet been standardized for synthesizing metamaterials, some of their potential applications have already been realized. Metamaterial antennas, for example, are already commercially available.
Metamaterial antennas display improved performance over alternative systems. Using metamaterials in an antenna helps to reduce its form factor, increase its directivity, and widen its tunable frequency.
Superlenses, two- or three-dimensional optical devices using metamaterials, have been shown to exceed the diffraction limit and might theoretically be able to achieve infinite resolution in imaging applications.
Also in the theoretical realm, some researchers have demonstrated that metamaterials could be used to create a science-fiction-like “cloaking” or “invisibility” device, although no practical demonstration has been recorded.
References and Further Reading
Brun, M., S. Guenneau, and A. B. Movchan (2009). Achieving control of in-plane elastic waves. Applied Physics Letters. Available at: https://doi.org/10.1063/1.3068491.
Guenneau, S., et al. (2007). Acoustic metamaterials for sound focusing and confinement. New Journal of Physics. Available at: https://doi.org/10.1088/1367-2630/9/11/399.
Oliveri, G., D. H. Werner, and A. Massa (2015). Reconfigurable Electromagnetics Through Metamaterials—A Review. Proceedings of the IEEE. Available at: https://doi.org/10.1109/JPROC.2015.2394292.
Parimi, P. V. et al. (2003). Imaging by flat lens using negative refraction. Nature. Available at: https://doi.org/10.1038/426404a.
Pendry, J. B. (2006). Negative Refraction. Contemporary Physics. Available at: https://doi.org/10.1080/00107510410001667434.
Shelby, R. A., R. Smith, and S. Schultz (2001). Experimental Verification of a Negative Index of Refraction. Science. Available at: https://doi.org/10.1126/science.1058847.
Zharov, A. A. et al. (2005). Birefringent left-handed metamaterials and perfect lenses for vectorial fields. New Journal of Physics. Available at: https://doi.org/10.1088/1367-2630/7/1/220.
Disclaimer: The views expressed here are those of the author expressed in their private capacity and do not necessarily represent the views of AZoM.com Limited T/A AZoNetwork the owner and operator of this website. This disclaimer forms part of the Terms and conditions of use of this website.