The use of gas sensors in detecting, monitoring, and evaluating combustion-related toxic and exhaust gases has attracted much interest. This technique finds practical application at both industrial and domestic levels as a tool for environmental protection and energy-saving in various industrial processes.
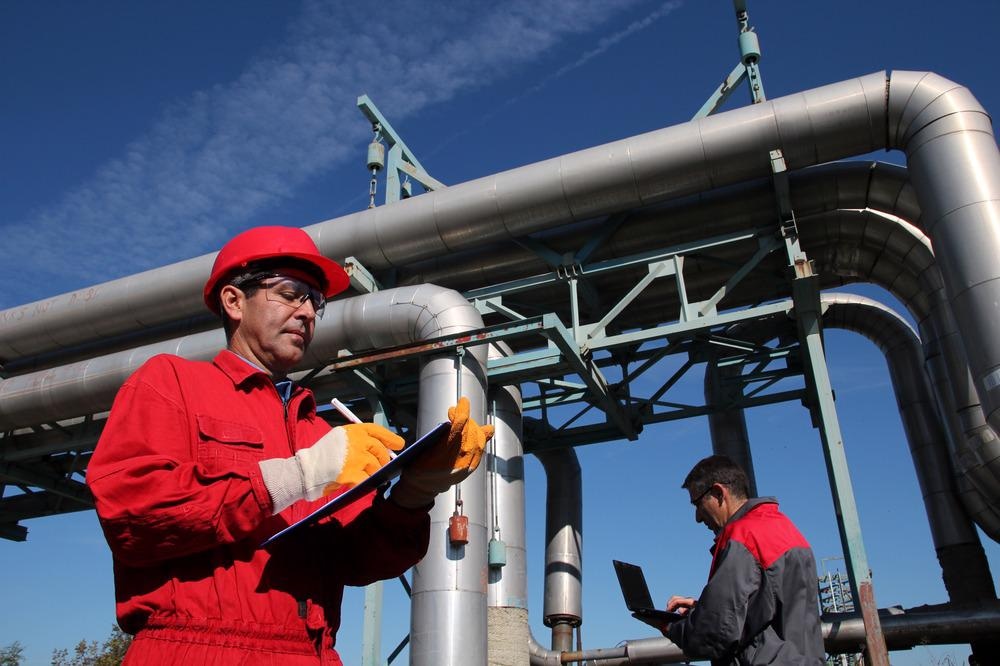
Image Credit: dgdimension/Shutterstock.com
Previously, many industrial processes have employed more complex gas detection techniques such as chemiluminescent analysis, gas chromatography/mass spectroscopy (GM/MS), and infrared spectroscopy.
These instruments offer fast response times and reasonable detection limits. However, they are relatively expensive, very bulky, inoperable in high-temperature environments, and require frequent maintenance. These factors as well as the need to integrate a gas sampling system fuel the search for better options.
Recent developments revolve around variations in sensing materials and detection methods. Based on this, gas sensors are classified according to their sensing methods into those that rely on their electrical properties and those based on other properties (optic, acoustic, calorimetry, and gas chromatography). Additional classification based on the sensing type includes paramagnetic, electrochemical, catalytic combustion, infrared absorption, and metal oxide semiconductor sensors. Categorizations based on detection methods include field-effect-transistors (FET), DC conductometric gas sensors, and photoluminescence (PL) based gas sensors.
Semiconductor metal oxide (SMO) gas sensors have attracted much research interest in the past decade due to their improved properties and applications. There has been a convergence of studies indicating that this type of sensor offers a plethora of benefits that can help mitigate the limitations of conventional variants of gas sensors.
The semiconducting direct thermoelectric gas sensor technique (DTEGs) involves a combination of SMO as the sensing material and applying a direct thermoelectric detecting approach. In this case, the surface of the SMO (carbon nanotube, 2D material, conducting polymer, metal oxide) acts as the gas sensor. It is exposed to the environment where it interacts, resulting in a change in measurable physical parameters of the SMO, such as permittivity and conductivity used for the detection and evaluation of gases.
The use of SMOs is dependent on their physical and chemical properties. Metal oxides are the most studied and most diverse class of SMOs owing to the ease of modifying their physical and chemical properties to achieve desired specifications for continuous improvements. These properties include surface area, chemical composition, morphology, structural defects, grain size, etc. The ability to control these parameters determines the overall quality of the gas sensor in terms of stability, resolution time, response time, working temperature, recovery time, and sensitivity.
This summary presents an overview of gas sensors, insights into its classification and types based on material components, detection methods, working principles, comparative functional advantages, applications, limitations, and an outlook into the most recent approaches.
Principle and Operation of Semiconducting Direct Thermoelectric Gas Sensors
DTEGs, like every thermoelectric couple, work on the principle of variation of its properties under specified conditions. In DTEGs, when the surface of the semiconductor material is hit by the gas molecules, the Fermi level of the semiconductor changes, causing a change in the material's conductivity. The position of the Fermi level is determined by the amount (concentration) of gas molecules that hit the sensor.
The thermo-voltage of the DTEGs system is used as an indicator, and because thermo-voltage is not dependent on the path traveled by the gas molecules, DTEGs are very resistant to damages occurring from abrasives in gas flow.
As the interaction of the gas molecules with the surface of the semiconductor material causes a change in the conduction activity of the material, the transducer, which is a component of the DTEGs, converts the physical parameter (conductivity) to an electrical parameter (resistance), thereby inducing a potential difference in the material which is then measured with a potentiometer and read as a gas sensing signal.
The semiconductor, the primary sensing material, is designed with a large and exposed surface area to sufficiently receive and interact with the gas molecules.
Applications of Semiconducting Direct Thermoelectric Gas Sensors
On a global scale, the application of gas sensors across various environmental, industrial, automotive, and medical spheres grows exponentially. The global market is estimated at 2 billion Euros.
In many industrial processes like oil exploration, semiconducting DTEGs have been employed in detecting gas leaks. Most fire alarms also use this approach to monitor flammable gas levels and detect smoke. This tool is also used in many homes and offices, where it helps provide a level of preventative and protective mechanisms against fire outbreaks.
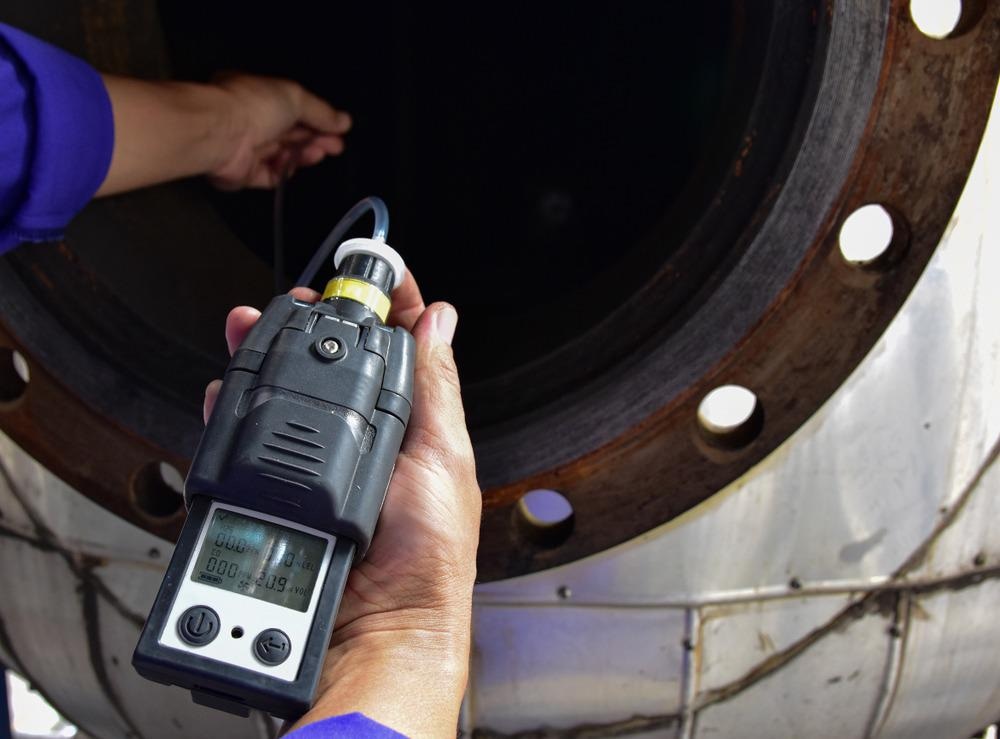
Image Credit: Tum ZzzzZ/Shutterstock.com
Recently, the use of DTEGs has spread to automobile manufacturing, where it is used for various purposes, including as a detector for engine emission control mechanisms.
Hydrogen detection is one of the most critical applications of gas sensors. Hydrogen gas (H2) is the lightest element known at standard temperature and pressure. It is also colorless, odorless, and tasteless. These characteristics make it virtually impossible to detect a hydrogen leak physically. However, the application of gas sensors helps to solve this challenge. The ability of gas sensors to detect hydrogen gas has made them useful in the aerospace industry, where hydrogen is used as a fuel and propellant.
Recent Innovations in Semiconducting Direct Thermoelectric Gas Sensors
Hybrid transducers have been developed with an improved temperature modulation technique. The advantage of this is improved accuracy, with the possibility of analyzing temperature difference signals and thermo-voltages using regression or Fourier's analysis. This approach ensures that the signal analysis filters out interfering voltages.
With a frequency modulation of 0.312 Hz, the DTEGs technology is not currently able to support many emerging technological applications. Thus, research is still underway to improve its frequency modulating ability. Intrinsic materials with enhanced sensitivity to improve DTEGs frequency modulation have been reported.
The use of ion-conducting materials as primary sensors in DTEGs is another ongoing research topic with great potential. Research shows that using ion-conducting material as the primary sensor can increase the selectivity of the technique owing to the unique conductivity of ions.
Final Overview and Future Developments
Proper monitoring of toxic and anthropogenically-induced combustion gases is critical for environmental monitoring and best industrial safety practices. Gas sensors have played an enormous role in providing an affordable, sensitive, and straightforward approach. The technique is a broad one that encompasses different types of sensor materials and detecting approaches to create an optimized method for practical measurements.
Although DTEGs have long been used for gas sensing, it is evident that it still does not provide all the answers required of a gas sensor. There is a need for continuous improvements in design to overcome the existing limitations.
More from AZoM: Considering the Behavior of Doped SrTiO3 Ceramics
References and Further Reading
Jack., C. (2000). Electrochemical Hazardous Gas Monitors: A Practical Guide to Selection, Operation, and Applications, pp: 28 – 34.
Frank, R., and Ralf, M. (2020). Chapter Eleven – Semiconducting Direct Thermoelectric Gas Sensors. Semiconductor Gas Sensors. 2nd Edition. Woodhead Publishing Series in Electronic and Optical Materials; Cambridge, UK. Pp: 347–381. https://www.sciencedirect.com/science/article/pii/B9780857092366500071
Frank, R. and Ralf, M. (2007). Direct Thermoelectric Gas Sensors: Design Aspects and First Gas Sensors. Sensors and Actuators B. 123(1) pp: 413 – 419.
https://www.sciencedirect.com/science/article/pii/S0925400506006186?via%3Dihub
Dey, A. (2018). Semiconductor metal oxide gas sensors: A review. Materials Science and Engineering B. 229(2018). https://doi.org/10.1016/j.mseb.2017.12.036
https://www.sciencedirect.com/science/article/abs/pii/S0921510717303574
Nikolic, M. V., Milovanovic, V., Vasiljevic, Z. Z., and Stamenkovic, Z. (2020). Semiconductor Gas Sensors: Materials, Technology, Design, and Application. Sensors (Basel, Switzerland), 20(22), 6694. https://doi.org/10.3390/s20226694
https://www.ncbi.nlm.nih.gov/pmc/articles/PMC7700484/
Saruhan, S., Fomekong, R. L. and Nahirniak, S. (2021). Review: Influence of Semiconductor Metal Oxide Properties on Gas Sensing Characteristics. Frontiers in Sensors (2). DOI=10.3389/fsens.2021.657931.
https://www.frontiersin.org/articles/10.3389/fsens.2021.657931/full
Guha, P. K., Santra S., Gardner J. W. (2020). Chapter Fourteen – Integrated CMOS-based Sensors for Gas and Odor Detection. Semiconductor Gas Sensors. 2nd Edition. Woodhead Publishing Series in Electronic and Optical Materials; Cambridge, UK. Pp: 465 –487. https://www.sciencedirect.com/science/article/pii/B9780081025598000148?via%3Dihub
Disclaimer: The views expressed here are those of the author expressed in their private capacity and do not necessarily represent the views of AZoM.com Limited T/A AZoNetwork the owner and operator of this website. This disclaimer forms part of the Terms and conditions of use of this website.