Over the past decade, efforts to achieve carbon-neutral operations have emphasized renewable and sustainable energy sources. These sources, however, often produce power inconsistently, making it challenging to integrate them into existing energy grids.
Energy storage systems are used to regulate this power supply, and Vanadium redox flow batteries (VRFBs) have been proposed as one such method to support grid integration.
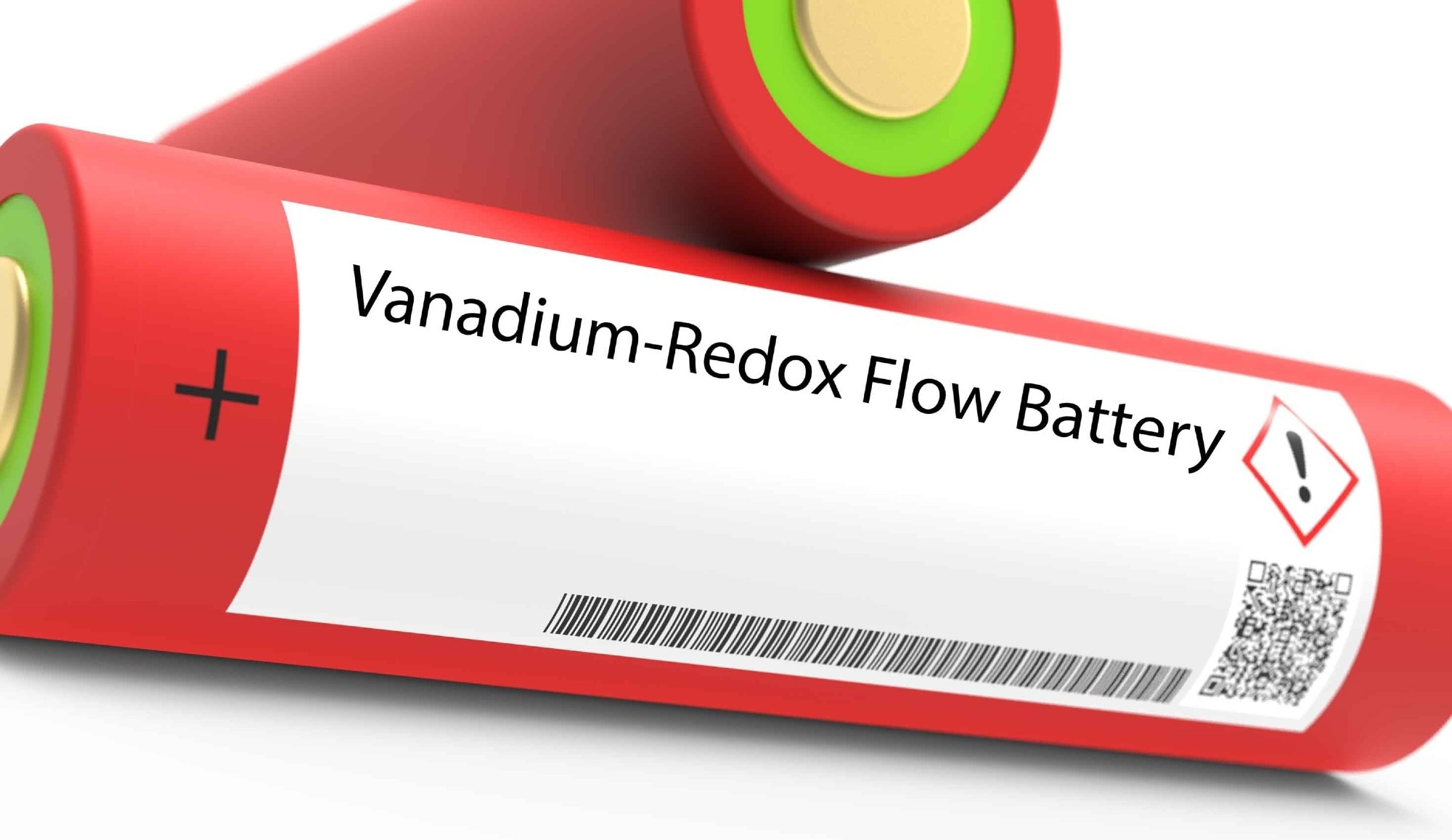
Image Credit: luchschenF/Shutterstock.com
Core Components of Vanadium Redox Flow Batteries
VRFBs include an electrolyte, membrane, bipolar plate, collector plate, pumps, storage tanks, and electrodes. Typically, there are two storage tanks containing vanadium ions in four oxidation states: V2+, V3+, VO2+ (V4+), and VO2+ (V5+).
Each tank contains a different redox couple.1 The positive side of the battery connects to the electrolyte and electrode associated with V4+ and V5+ ions.
Electrolyte Composition and Performance Characteristics
The use of the same active species on both sides minimizes capacity losses from cross-contamination. A standard VRFB can store about 20–30 Wh/L of electrolyte, with the output voltage typically around 1.3V.2
The electrolyte concentration determines how much is used. V2O5 is considered cost-effective for electrolyte production, while VOSO4 offers more flexibility for adjusting concentrations.
Download your PDF copy now!
How VRFBs Work: Operating Principle and Cell Reactions
Electrolytes are pumped through two separate half-cells and returned to the storage tanks. Each half-cell consists of an electrode and a bipolar plate, separated by a membrane that allows selective ion exchange. This arrangement forms a single cell, and several cells are stacked using shared bipolar plates.
At the positive half-cell, VO2+ reacts with H⁺ and an electron to produce VO2+ and water. At the negative half-cell, V2+ is oxidized to V3+, releasing an electron. The overall reaction is:
VO2+ + V 2+ + 2H + ⇌ VO 2+ + V 3+ + H2O
Multiple stacks of VRFBs are connected electrochemically to enable energy storage for large-scale applications. In a typical setup, the stacks and cells receive a continuous supply of electrolyte in parallel, which helps maintain a stable concentration of redox-active ions across the system.
During charging, electrons move through the bipolar plates from the positive to the negative side, while hydrogen ions (H⁺) pass through the membrane in the same direction.3 This electrochemical process allows VRFBs to support energy needs in both industrial and residential settings.
How the Vanadium Redox Flow Battery "VRFB" Works
VRFBs: The Good, the Bad, and the Practical
VRFBs provide design flexibility due to the use of liquid electrolytes, which can be stored in tanks of various shapes and sizes. The separation of power and energy capacity allows for independent scaling, which can be useful in industrial applications.
These batteries also tend to have a longer cycle life than conventional batteries, as the liquid electrolytes degrade more slowly over time, even with some degree of crossover. The separation of the energy storage (tanks) and power generation (cell stacks) components enables more flexible system layouts.
For example, tanks can be installed in underground or less space-constrained areas, while cell stacks can be located where heat management is more efficient. This arrangement can simplify cooling system design and potentially reduce associated costs.4
However, despite these design advantages, VRFBs also face notable limitations, particularly when it comes to mobile applications. Their relatively low power and energy densities make them less practical in compact or portable systems. Power densities are typically around 15 W/cm2 in the cells, 100 W/cm2 in the stack, and approximately 25 Wh/kg in the electrolyte solutions.
These characteristics often necessitate the use of large electrolyte tanks, limiting their application in compact or mobile systems. Additionally, electrical efficiency can be reduced by the presence of shunt currents within the battery system.5
Another challenge is the unintended transfer of vanadium ions and water across the membrane, including diffusive and electro-osmotic crossover effects. Water imbalance between the battery compartments can result in the precipitation of vanadium salts, which negatively affects performance.
Managing this imbalance requires careful system control. The relatively high viscosity and flow requirements of the electrolyte also contribute to increased pressure drops and higher pumping power, which impacts overall system efficiency.6
Vanadium, the key active material in VRFBs, is primarily used in the steel and chemical industries. For example, in Germany, about 90 % of vanadium consumption is for steel production. This demand limits the availability of vanadium for battery production and contributes to higher material costs.
Additionally, the number of vanadium mines is smaller than resources such as lithium, resulting in supply constraints. There are also concerns related to vanadium toxicity, and health, safety, and environmental (HSE) regulations can restrict its handling and usage.7
These technical and material challenges, combined with regulatory hurdles, initially limited industry interest in VRFBs. At the same time, lithium-ion batteries (LIBs) gained market traction and became the dominant technology for energy storage in consumer electronics and electric vehicles, further slowing the commercial adoption of VRFBs.
Curious About the Difference Between Lithium and Lithium-Ion Batteries? Read the Full Explanation Here.
How VRFBs Are Being Used
Redox flow batteries, including VRFBs, are well-suited for stationary energy storage applications where power output and energy capacity are designed to remain in a fixed ratio. Their operational safety, modular scalability, and high cycle life make them a viable option for such use cases.8
VRFBs can support the integration of renewable energy sources into large power grids by helping to stabilize supply, manage peak loads (peak shaving), and contribute to grid reliability.
In wind and hydroelectric systems, VRFBs can assist with power smoothing by absorbing short-term fluctuations in output without significantly impacting the overall performance of the generation system. This helps ensure more stable power delivery to the grid.
Additionally, VRFBs can be integrated with the DC link in systems such as wound rotor synchronous generator (WRSG)-based wind turbines. This configuration may improve the system's ability to handle low-voltage events, thereby enhancing grid stability during voltage disturbances.9
What’s Next for VRFBs?
For VRFBs to become more viable for large-scale commercial applications, key technical challenges must be addressed, particularly those related to membrane and electrode performance.
Developing low-resistance membranes and more efficient electrodes can help reduce cell resistance and improve overall system efficiency. Additionally, reducing the frequency of regeneration cycles (e.g., from once per year to once every five years) could lower maintenance costs.
Projections suggest that increasing round-trip efficiency from approximately 75 % to 85 %, and extending battery life to around 14,600 cycles (equivalent to 40 years), could significantly improve the commercial feasibility of VRFBs in long-duration energy storage (LDES) projects.10
Progress in areas such as advanced materials research, cost optimization, supportive policy frameworks, and targeted government incentives may contribute to the broader adoption of VRFBs in the energy storage sector over the coming decade.
References and Further Reading
- Gundlapalli, R. et. al. (2018). Stack design considerations for vanadium redox flow battery. INAE Letters, 3(3), 149-157. Available at: http://dx.doi.org/10.1007/s41403-018-0044-1
- Zhang, H. et. al. (2017). Redox flow batteries: fundamentals and applications. CRC Press. Available at: https://www.doi.org/10.5772/intechopen.68752
- Lourenssen, K. et. al. (2019). Vanadium redox flow batteries: A comprehensive review. Journal of Energy Storage, 25, 100844. Available at: https://doi.org/10.1016/j.est.2019.100844
- Cunha, Á. et. al. (2015). Vanadium redox flow batteries: a technology review. International Journal of Energy Research, 39(7), 889-918. Available at: https://doi.org/10.1002/er.3260
- Olabi, A. et. al. (2023). Redox Flow Batteries: Recent Development in Main Components, Emerging Technologies, Diagnostic Techniques, Large-Scale Applications, and Challenges and Barriers. Batteries. 9(8). 409. Available at: https://doi.org/10.3390/batteries9080409
- Guarnieri, M. et. al.(2016). Vanadium redox flow batteries: Potentials and challenges of an emerging storage technology. IEEE Industrial Electronics Magazine. 10(4). 20-31. Available at: https://doi.org/10.1109/MIE.2016.2611760
- Ciotola, A. et. al. (2021). The potential supply risk of vanadium for the renewable energy transition in Germany. Journal of Energy Storage, 33, p.102094. Available at: https://doi.org/10.1016/j.est.2020.102094
- Girschik, J. et. al. (2021). Redox flow batteries: stationary energy storages with potential. Chemie Ingenieur Technik, 93(4), pp.523-533. Available at: https://doi.org/10.1002/cite.202100001
- Aluko, A. et. al. (2023). A review on vanadium redox flow battery storage systems for large-scale power systems application. IEEE Access, 11, 13773-13793. Available at: https://doi.org/10.1109/ACCESS.2023.3243800
- Poli, N. et. al. (2024). Techno-economic assessment of future vanadium flow batteries based on real device/market parameters. Applied Energy, 362, 122954. Available at: https://doi.org/10.1016/j.apenergy.2024.122954
Disclaimer: The views expressed here are those of the author expressed in their private capacity and do not necessarily represent the views of AZoM.com Limited T/A AZoNetwork the owner and operator of this website. This disclaimer forms part of the Terms and conditions of use of this website.