Controlling the configuration of graphene oxide (GO) phases, or rather their smaller synthetic analogs, graphene (oxide) quantum dots (GOQDs), is crucial for any of their commonly used applications, as stated in the Scientific Reports Journal. This includes highly ordered nanomaterials arrangements for thin-film or outer layer applications, distributed nanoparticles for matrix composites, and three-dimensional porous structures for hydrogels.
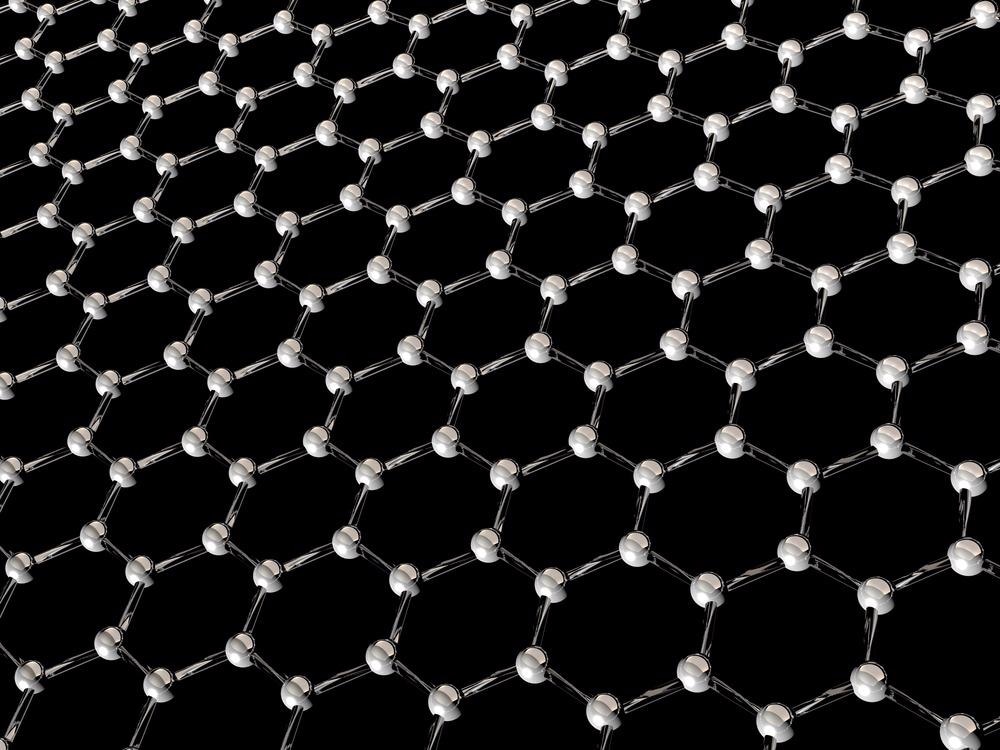
Study: Principles governing control of aggregation and dispersion of aqueous graphene oxide. Image Credit: Jarek_Sz/Shutterstock.com
The morphology of GO flakes in aqueous settings is influenced by extrinsic variables such as pH and coexisting cations, as well as the chemical properties of the flakes themselves. Because of GO's unique hydrophilic, heat, and electrical characteristics, there is a lot of interest in leveraging the chemical properties of GO flakes to produce self-assembled GO structures with application-specific geometries.
Boosting GO for Widely Used Applications
To boost the mechanical modulus and strength of films and fibers, high alignment and strong contacts between the flakes are required. Porous structures, on the other hand, are preferred for energy storage devices such as supercapacitors and hydrogels.
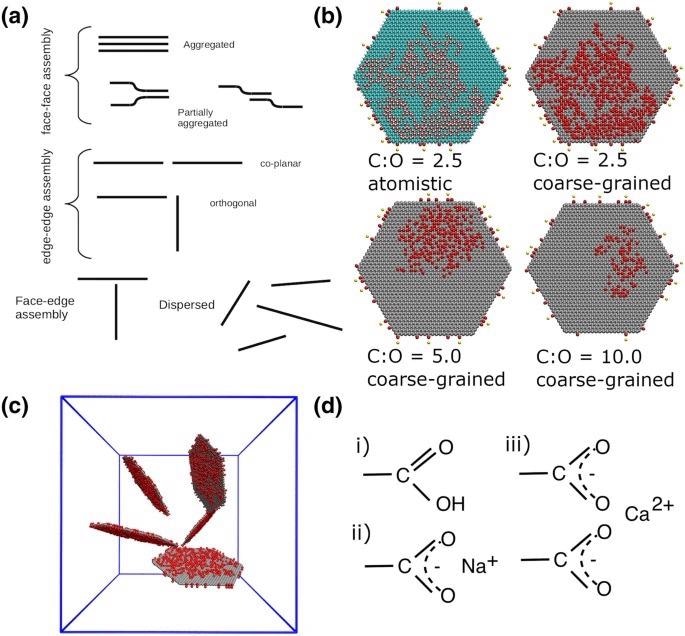
(a) Schematic diagram displaying the different morphologies and aggregation interaction modes formed by the graphene (oxide) flakes. The flakes at the top right (b) are examples of GO sheets oxidized to different extents. The first flake shows the GO flake with a C:O ratio of 2.5 at the atomistic level. In this example, the carboxylic edge groups are deprotonated with a calcium counterion. The color scheme for atomistic systems is: carbon is cyan, oxygen is red, hydrogen is white, calcium is yellow and sodium is blue. The remaining flakes are shown at the coarse-grained level. The red circles represent the oxidized hydroxyl groups and edge carboxylic groups, and grey represents graphitic carbon atoms. The diameter of each flake is 10 nm. (c) shows the dispersed initial configuration (water not shown). There are five flakes within each simulation cell. The solid blue lines are the periodic boundaries, with lattice parameters of approximately 18nm. d) shows the different carboxylic edge groups considered in this study, (i) protonated \ neutral (i.e low pH), (ii) deprotonated with sodium counterions and (iii) deprotonated with calcium counterions. All atomistic and coarse-grained visualizations in this and subsequent figures were created using VMD Version 1.9.248,49. Image Credit: Suter, J., and Coveney, P., Scientific Reports Journal
Dispersion is necessary for some purposes; aggregation and the resulting loss in surface area have been described as major components in lowering GO's protein-binding capabilities, which has consequences in the pharmaceutical industry.
GO is also being considered as a possible adsorbent for the removal of environmental pollutants, such as radionuclides, by the creation of nanoparticulate aggregation.
Another Form of GO, Graphene-Oxide Quantum Dots (GOQDs)
Graphene-Oxide Quantum Dots (GOQDs), which are a scaled-down form of GO, have recently sparked a lot of attention. GOQDs have demonstrated multiple benefits in bioimaging, bio- and metal sensors, photovoltaic cells, and photocatalysts related to edge and quantum hall effects. Because GOQDs contain a higher fraction of edge sites than GO, they are more hydrophilic whilst maintaining the same amphiphilic characteristics as GO10. Photoluminescence is one of the most important applications of GOQDs.
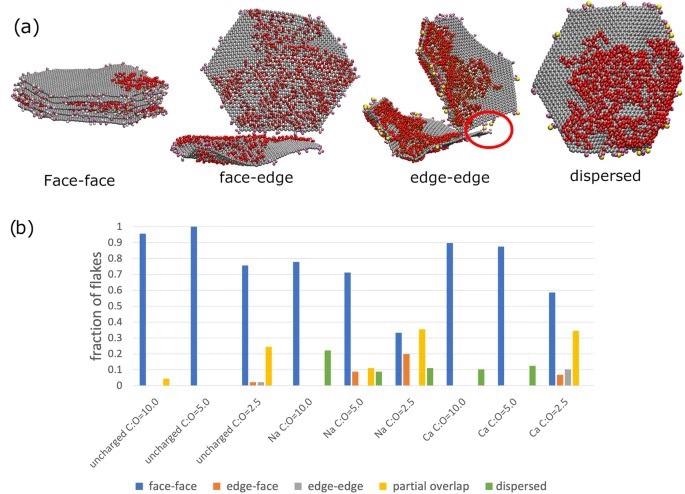
Aggregation interaction modes present in graphene oxide flakes in aqueous solution. (a) Snapshots from the coarse-grained simulations illustrating the relevant binding mode. The red circle on the edge-edge binding mode snapshot highlights how a Ca2+ is acting as a ion bridge and facilitating the edge-edge binding mode. The colour scheme is the same as Figure 1. In (b), we display the percentage of flakes in each binding mode for the different systems in our study. The different binding modes (“face-face”, “partial”, “edge-edge” and “face-edge” are schematically illustrated in Fig. 1). Flakes that are not bound are classed as dispersed. Image Credit: Suter, J., and Coveney, P., Scientific Reports Journal
Both GO and GOQDs have been shown to exhibit domains of oxidized and graphitic areas in ultrahigh-resolution TEM or transmission electron microscopy pictures rather than a homogenous distribution of oxidation sites over the GO surface.
These areas can either serve as building blocks for self-assembling 3D designs or serve as the catalyst for the production of undesirable aggregated architectures. Controlling secondary interactions like electrostatic and hydrogen bonding is thus essential, either by modifying the chemical structure of the flakes or by changing external parameters like pH or the presence of counterions.
Aggregation of GO in Water
Several variables have been discovered to influence the aggregation of GO in water in studies. The great solubility of GO in water, in which a huge number of oxygen-containing functional groups are deprotonated, is well recognized.
The oxygen-containing functional groups are protonated at low pH, reducing the charge on the surface of GO. Large-scale visible flocculent aggregation develops when electrostatic repulsion is reduced. The negative charge is screened by increasing the ionic strength and osmotic pressure of the aqueous solution, and the essential coagulation concentration is decreased.
Molecular modeling is a great tool for studying GO flakes in polymeric substrates because it allows researchers to alter the structure and composition of GO, evaluate thermodynamically stable morphologies of GO, and observe dispersion and aggregation dynamics with a high level of realism.
Researchers may check to see if the aggregation modes seen in simulations are the same as those hypothesized, such as edge-to-edge and face-to-face binding. Users can examine the equilibrium between interactions with molecules of water, ion, and other flakes by evaluating the interactions existing in our aggregated GO flakes in atomic detail.
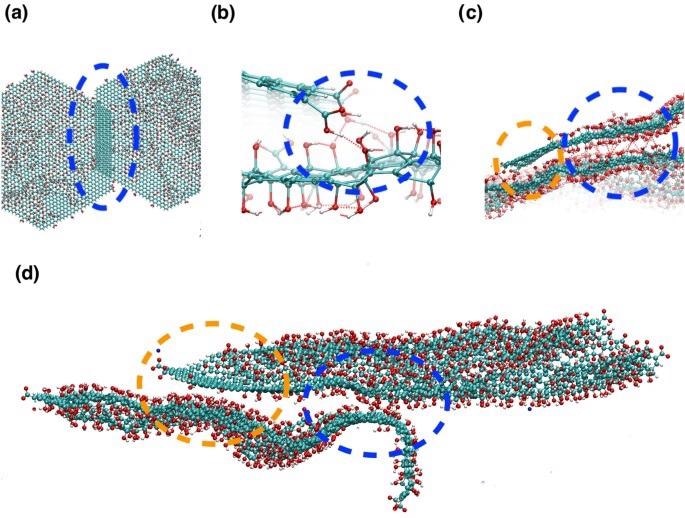
Snapshots from the atomistic simulations at 300K after the simulated annealing run, illustrating the different partial face-face binding modes observed for GO with a C:O ratio of 2.5. (a)–(c) are snapshots for uncharged (i.e. low pH GO), (d) is a snapshot for charged GO flakes with Na+ ions. The different binding modes are highlighted by dashed ovals and circles. (a) The flakes have overlapped due to π–π stacking interactions between sp2 carbon atoms. (b) The protonated carboxylic acid atoms form hydrogen bonds with hydroxyl groups on overlapping flakes. Hydrogen bonds are shown by red dashed lines, defined by the distance between oxygen and hydrogen atoms < 4.5 Å and acceptor oxygen–donator oxygen–hydrogen atom angle < 40°. (c) Blue circle: hydroxyl groups form hydrogen bonds with hydroxyl groups on overlapping flakes. Orange circle: sp2 carbon atoms are interacting with an area of oxidised graphene oxide on an adjacent sheet, via van der Waal’s interactions. (d) Binding modes for two charged GO sheets with Na+ ions. The orange circle highlights interactions between a sp2 carbon atoms and hydroylated regions on an adjacent flake, the blue circle shows hydrogen bonded interactions between the flakes. The lower flake has become wavy and contorted to keep apart the charged carboxylate groups. Image Credit: Suter, J., and Coveney, P., Scientific Reports Journal
GO and GOQD Aggregation
Understanding the link between flakes structure and composition is crucial for aqueous graphene oxide and graphene oxide quantum dot systems to work optimally. Our simulations, which included realistic subdomains of oxidized and non-oxidized areas, revealed that these domains are critical for GO and GOQD aggregation. We've seen a number of interactions among aggregated sheets, including high-density oxidation areas establishing hydrogen bonds with surrounding flakes and graphite-like stacking in sp2 carbon regions.
Low pH allows the carboxylic acid edge units to protonate, causing the flakes to aggregate in a face-to-face binding mode, as demonstrated in the experiment and corroborating the reduction in electrostatic interaction reported in DLVO investigations.
The degree of oxidation on the surface of the formed flake determines the shape of the formed flake at low pH; highly oxidized GO forms planar aggregates of overlapping flakes that bind through a number of various interactions. Hydrogen connections between hydroxyl groups on separate sheets, sp2 region overlap, and connections between carboxyl acid groups and hydroxyl groups on various flakes are all examples.
As demonstrated in experiments and anticipated from DLVO research, a high pH with sodium counterions promotes the creation of stable dispersions of one or two flakes. Notwithstanding the electrostatic repulsion of the flakes, hydrogen bonding among regions of high hydroxyl density and van der Waals contacts between sp2 or oxidized domains are significant enough to induce certain highly oxidized GO flakes of the same charging to assemble into tactoids comprised of two flakes.
References
Suter, J., and Coveney, P. (2021). Principles governing control of aggregation and dispersion of aqueous graphene oxide. Published: 17 November 2021. https://www.nature.com/articles/s41598-021-01626-3
Disclaimer: The views expressed here are those of the author expressed in their private capacity and do not necessarily represent the views of AZoM.com Limited T/A AZoNetwork the owner and operator of this website. This disclaimer forms part of the Terms and conditions of use of this website.