Writing in the journal Applied Surface Science, a team of researchers from the Air Force Research Laboratory has analyzed the electronic structure and electron transport properties of patterned graphene using first-principles computational modeling. The paper is currently in the pre-proof stage of publication.
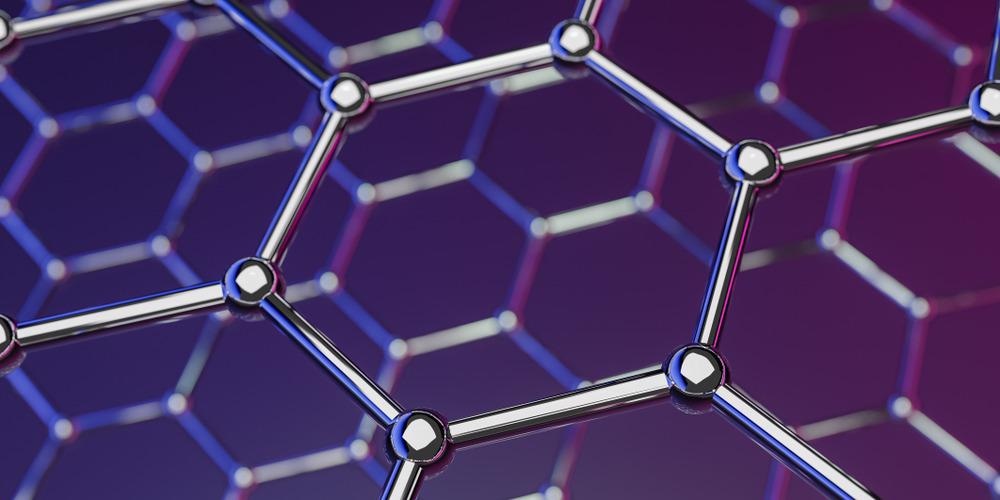
Study: Patterned graphene: Analysis of the electronic structure and electron transport by first principles computational modeling. Image Credit: Production Perig/Shutterstock.com
Carbon-Based Nanomaterials
Carbon-based nanomaterials have been widely investigated for use in applications such as microelectronics, renewable energy harvesting and storage, wearable tech, and biomedical devices. Semi-metallic graphene, whilst it possesses several interesting properties, has limitations that hinder its use in field-effect transistors.
To overcome the limitations of this nanomaterial, graphene nanoribbons have been widely evaluated for use in RF communication, wearable electronics, and biological and chemical sensing. These nanomaterials are one-dimensional strips of graphene. Studies have indicated that, theoretically, armchair graphene nanoribbons possess high on-off ratio values, high carrier mobility, can achieve sizeable bandgaps, and sufficient cut-off frequency. These properties make graphene nanoribbons attractive candidates for thin-film electronics.
However, a major drawback of graphene nanoribbons is the presence of edge defects. Studies investigating the materials using a tight-binding model have indicated that a single edge defect can possibly induce quasi-localized states. This can cause zero-conductance dips in the material, affecting its electrical performance. To ensure good thermal conductivity, graphene nanoribbons must possess smooth edges, but synthesis of these nanomaterials with defined edges remains challenging.
Synthesis methods for creating narrow, smooth-edged graphene nanoribbons are limited, such as bottom-up organic synthesis and lateral heteroepitaxy. Reduced graphene oxide can be used as a material, but this is dependent on the reduction route during synthesis. Recent work has tuned the bandgap of reduced graphene oxide, but randomly distributed defects still occur which can negatively impact the efficiency of electron transport.
Recently, there has been research focus on patterned graphene. This nanomaterial is composed of conductive graphene regions, with alternated functional regions also existing in the material.
The Research
Building on previous research by the authors which developed patterned graphene, the current research by the scientists at the Air Force Research Laboratory in Ohio has analyzed the electronic properties and electron transport behavior of this novel nanomaterial using computational modeling.
Synthesizing patterned graphene is a complex process. An electron beam is applied to graphene in the presence of water vapor in an environmental scanning electron microscope. This generates radiolyzed moieties, with reactive oxygen species interacting with the graphene’s lattice to form functionalized defects. Notwithstanding the complexity of the process, the authors have stated that the patterning of alternating pristine and defective strips could be useful for applications such as biosensing.
Moreover, gap opening can be facilitated by patterned graphene, and its use could overcome several challenges with current graphene nanomaterials for use in nano-electronic devices such as controlled edge termination and electrode contact resistance. The authors have stated that techniques such as support-free transfer and UV/ozone treatment which preserve the material’s intrinsic properties could be employed to transfer the material onto dielectric substrates.
More from AZoM: How Do We Recycle Semiconductors?
Density functional theory calculations were used by the researchers to investigate patterned graphene’s electronic structures and electron transport. Analysis of the material’s electronic structures indicated a relationship between bandgap and the width of the strip. Dirac electrons were confined in the graphitic strips. An intriguing observation was the formation of artificial graphene nanoribbons by the absorption of oxygen atoms onto carbon-carbon bonds.
Transmission paths in the modelling demonstrated confinement of electrons within the graphene strips with agreed with the confinement in the electronic structures of the patterned graphene. Weak electron scattering was demonstrated at the functionalized graphene/graphene interface in the material. This was due to electrons injected from the graphene side to the interface being scattered back to the graphene strip.
The authors also investigated electron transport in devices which possess different configurations of electrode-central regions to study the effect of realistic electrodes. Amongst their observations, the authors found that device optimization of graphene electrodes indicated smooth contact between graphene and patterned graphene with minimal bond distortions.
In Summary
The authors have evaluated the electronic structures and electron transport properties of patterned graphene, which is composed of graphene strips and alternated functionalized graphene strips, using first principle density functional theory calculations. The results of the study demonstrated that patterned graphene can be considered an array of artificial graphene nanoribbons. These structures display uniform separation, edge, and width. Their bandgap is highly tunable.
Using device engineering, the patterned graphene strips can be placed and integrated into devices. Contact resistance of the patterned graphene was evaluated and demonstrated promising potential for use as an electrode material. Overall, the results of the study have indicated that patterned graphene could overcome current challenges with using graphene nanoribbons with edge defects. The authors noted a relatively small deterioration at the edge of defective regions in patterned graphene.
Further Reading
Jiang, J et al. (2022) Patterned graphene: Analysis of the electronic structure and electron transport by first principles computational modeling [pre-proof] Applied Surface Science 152953 | sciencedirect.com. Available at: https://www.sciencedirect.com/science/article/abs/pii/S0169433222005232
Disclaimer: The views expressed here are those of the author expressed in their private capacity and do not necessarily represent the views of AZoM.com Limited T/A AZoNetwork the owner and operator of this website. This disclaimer forms part of the Terms and conditions of use of this website.