The term liquid crystal (LC) is a very common term; people know it from liquid crystal displays of digital clocks and watches dating back to the 1970s. Since then, LCs have substituted nearly all traditional displays for portable devices such as personal computers, toys, cell phones, and more recently, for televisions.
However, have you ever heard of liquid crystal polymers (LCPs)? Although these unique molecules have been adapted to a wide range of applications, they recently attracted significant attention from a new sector: For almost 20 years, the medical sector has researched the development of catheters, which are usually built with metal componentry and can be employed for magnetic resonance imaging (MRI) medical procedures. However, MRI prevents the use of metals. This article discusses LCPs in detail and shows how ZEUS leveraged the exceptional characteristic of LCPs to create a sophisticated monofilament fiber for constructing a catheter that is fully compatible with MRI.
Discovery
In spite of their more recent commercialization, the discovery of liquid crystals dates back to the late 19th century to two European scientists, Austrian German Otto Lehmann and Friedrich Reinitzer. Initially analyzed by Reinitzer and validated by Lehmann a short time later, these scientists noted that when solid cholesteryl benzoate was heated and cooled, it underwent multiple phase transitions. On heating, the solid initially became a hazy liquid but when it was continuously heated it became clear. However, the opposite effect was noted when the heated clear liquid was allowed to cool down: it was first clear, then became a hazy liquid, and finally turned into a white solid. For Lehmann and Reinitzer, the troubling question was the apparent two liquid states of the cholesteryl benzoate, i.e., clear and hazy. (Solids typically melt at a narrow melting point range of just one degree or two and color and phase transitions are instant. For instance, pure ice melts exactly at 32 °F). In fact, Lehmann and Reinitzer had explained two different melting points for the same material – a phenomenon that was not known before. When observing the hazy liquid under a microscope, Lehmann described viewing crystallites – formations of multiple tiny crystallites with irregular borders. On further heating, the hazy liquid became clear and the crystallites disappeared. Lehmann discovered that this initial intermediate fluid appeared to be crystalline in nature which he implied was a new state of matter. He later termed his discovery as a liquid crystal, and the examination of two melting points would become essential to its identification.
What are Liquid Crystals?
What Lehman, Reinitzer, and others had discovered was a novel state of matter that lies somewhere between a true solid and a liquid. Thus, this new in-between phase of matter became to be known as a mesophase, and the molecules inside it were named mesogens. These organic molecules, which were small to moderate in size, arrange themselves in different degrees of organization along an axis known as the director, as shown in Figure 1. The director typifies the longer range order of the liquid crystal, but not all mesogen molecules take part in this ordering thus giving the LC unique behavior as neither a liquid nor solid. In addition, researchers observed that the positional orientation of the mesogen along the director was a basic element and led to different crystal formations.
Earlier in 1922, Georges Friedel, a French mineralogist, suggested a classification system based on mesogen alignment and accounting for the varying behaviors of LC types. Several basic crystal forms were described by Friedel that have since been explained in great detail. Here, smectic and nematic liquid crystal structures are of particular interest (Figure 2). While nematic liquid crystal molecules retain their directional orientation, they do not maintain some freedom of movement within the LC. Smectic mesogens are arranged in such a way that their principal axes lie parallel with their centers of mass in one plane. Smectic LCs exhibit directional and positional order. This orientation of molecules affects the LCs’ material properties such as magnetic, optical activity, and electrical properties; this effect is known as anisotropy. However, for gases or liquids, which show complete disorder or disorientation of molecules in those states, they show isotropy and their properties are also isotropic (Figure 1). A second defining characteristic of LCs is anisotropy.
.jpg)
Figure 1. Diagram showing the arrangement of mesogen molecules along the director within a substance. (A) Liquid (isotropic) and (B) crystalline (solid, anisotropic) forms.
.jpg)
Figure 2. Diagram showing the arrangement of mesogen molecules within liquid crystal types. Liquid crystal forms exhibit directional alignment of their molecules and are anisotropic. (A) Nematic liquid crystal showing orientational order, (B) smectic A liquid crystal form, and (C) smectic C liquid crystal form. B and C possess orientational and positional order.
Liquid Crystal Polymers
With additional research, scientists later came to know that LCs are also formed from more complex polymeric molecules. As their name indicates, liquid crystal polymers or LCPs are derived from LCs to exploit their remarkable properties. These molecules contain repeated monomer units, which are connected to form extended chain-like molecules. Most LCPs are characterized by a common basic structure that consists of three parts: a hydrophobic flexible linker on one end, a hydrophilic moiety on the other end, and a rigid mesogen core (Figure 3). These chain structures form LCPs by aggregating and self-aligning, just like single mesogen molecules do to form LCs. However, LCPs’ chain-like nature considerably affects improved intermolecular interactions leading to profound effects on LCP behavior, unlike simple (non-polymeric) LCs.
.jpg)
Figure 3. Representative example LCP monomer showing hydrophobic and hydrophilic regions, mesogen, and the flexible linker region.
LCPs and Thermoplastics
In this study, LCPs and their potential in thermoplastic applications are of particular interest. Many thermoplastics such as polystyrene (PS), polyvinyl chloride (PVC), polyethylene (PE), polytetrafluoroethylene (PTFE), polypropylene (PP), and Kevlar® (Figure 4) are well known. These thermoplastics are all simple hydrocarbon chains, and except for polystyrene, do not contain ring components. In fact, ring substituents are another feature that is common to LCPs, and polystyrene monomer is often incorporated into blends to create LCPs. LCPs, in their flow state during processing, distinguish themselves from standard thermoplastics because they have considerable crystallinity. This partial crystalline state of LCPs imparts these plastics with many unique properties such as toughness, exceptional strength, chemical resistance, and high temperature. Today, LCP plastics can be found in a wide range of applications, including food containers, laser beam deflectors, and aerospace parts.
.jpg)
Figure 4. Representative thermoplastic polymer monomers. Of these, polystyrene is the most frequently incorporated into an LCP.
Commercialization
For manufacturability, most commercial LCPs include p hydroxybenzoic acid (p-HBA) as one of its monomers as shown in Figure 5. Processing involves many condensation techniques and usually incorporates other monomers such as hydroquinone (HQ), bisphenol (BP), terephthalic acid (TA), 2,6 naphthalenedicarboxylic acid (NDA), isophthalic acid (IA), 6 hydroxy-2-naphthoic acid (HNA), etc. to facilitate synthesis (Figure 6). In addition, the two melting points of LCs – and of LCPs – offer a broader processing window for these unique polymer plastics. For instance, poly(4-hydroxybenzoic acid) is a high-melt polyester that forms from the condensation of p-HBA monomers, and then polybisphenol-A-teraphthatlate is produced from bisphenol A (BP) and terephthalic acid (TA), as depicted in Figure 5. In fact, LCPs are amenable to blend processing with polyester building blocks such as polyolefins and polycarbonate as well as common fillers such as carbon and fiberglass. The ability to vary the composition of LCP expands both their range of properties and their potential applications.
.jpg)
Figure 5. Example polyarylate polymer condensation reactions. (A) Polymerization of p-HBA monomers yield poly(p-hydroxybenzoic acid) polymer, and (B) Teraphthalic acid (TA) and bisphenol A monomers yield polybisphenol-A-teraphthalate polymer (monomeric unit shown).
.jpg)
Figure 6. Common additives which can be combined with p-HBA for LCP syntheses and processing.
ZEUS LCP Monofilament
Due to the unique material properties that are possible with LCPs, ZEUS has developed an LCP polymer as an extruded monofilament fiber. This product was first aimed towards medical applications, but it can potentially be used in a wide range of applications. A unique method is used to produce ZEUS’ LCP monofilament. This method involves the condensation of HNA and p HBA which results in a copolymer polyester called poly(4 hydroxybenzoic acid-co-6-hydroxy-2-naphthoic acid), as illustrated in Figure 7. This copolymer polyester contains high-continuity chains and forms an arbitrary melt-processable (thermotropic) material.
In terms of structure, this LCP fiber contains rod-like mesogens which form nematic LCs. During extrusion, these self-aligning molecules are longitudinally arranged in the flow direction leading to improved strengthening of the material, as shown in Figure 8. The exclusive structural attributes combined with proprietary processing result in a LCP fiber with unique physical and mechanical properties.
.jpg)
Figure 7. LCP condensation synthesis from p-hydroxybenzoic acid and 6-hydroxy-2-naphthoic acid.
.jpg)
Figure 8. Representation of LCP alignment during extrusion and the effect on fiber strength. Adapted from: Holliday L: The Stiffness of Polymers in Relation to Their Structure. In: Structure and Properties of Oriented Polymers. Edited by Ward IM. Dordrecht: Springer Netherlands:1975:242-263).
MRI-Compatible Catheters and LCP Monofilament
Since its development in the 1970s, MRI has attracted significant interest for expanding its use in medical diagnostics. However, the most major barrier to this objective is that MRI prevents the use of metals in devices, including catheters, which would be employed under the MRI field. In such situations, using an X-ray for soft tissue visualization procedures requiring catherization is the standard practice because the metal reinforcing braiding is used in catheter construction. An apparent result of X-ray is that it exposes both clinicians and patients to ionizing radiation. Instead, there is a need for catheters which can be utilized even under MRI to allow better quality and safer and visualization procedures.
In order to deal with the challenge of MRI-compatibility and catheterization, several companies have attempted to develop catheters with a variety of non-metal braiding components. Despite the fact that this effort has been ongoing for almost 20 years, it has created only mixed results. In this context, ZEUS LCP monofilament fiber is being employed as catheter reinforcement braiding instead of stainless steel and other metal and fiber braiding (Figure 9). The aim of this LCP braiding is to give the structural stiffness required by clinicians, while enabling the catheter to retain the desired characteristics such as flexibility for distal end deflectability. In addition, these catheters should have the rigidity and strength for pushability to navigate the human vasculature.
.jpg)
Figure 9. (A) Catheter construction components showing how LCP monofilament catheter braiding is applied as structural reinforcement. (B) Enlargement of LCP monofilament fiber braiding after being applied to the catheter.
As a substitute for traditional metal catheter braiding such as stainless steel wire, ZEUS LCP monofilament was compared to Type 304 V stainless steel (SS) wire – a type of braiding wire generally used in the construction of catheter – for a number of relevant characteristics. For these comparisons, spring tempered, annealed, and 1/4 hard SS wire of rectangle and round profiles were employed (Table 1). The LCP fiber showed excellent tensile strength to the annealed round as well as 1/4 hard rectangle SS wires, and also exhibited an elongation at break similar to the SS round and 1/4 hard rectangle wires. Further, the considerable tensile modulus of the LCP fiber of 75.0 GPa indicates that the LCP possesses the preferred stiffness needed for catheter shaft support. These traits result in better stiffness and torsional properties for the catheter and at the same time retain a degree of flexibility for catheter manipulation – highly attractive traits for these types of devices.
Table 1. Properties comparison of Zeus LCP monofilament fiber and 304 V stainless steel wire. Averages were calculated from five replicate trials. Tensile strength was measured according to ASTM D2256 guidelines. Ksi = 1000 x psi; GPa= 109 x pascal; mm = millimeter.
|
Zeus LCP Fiber |
SS wire (spring tempered) |
SS wire (annealed) |
SS wire (spring tempered) |
SS wire (1/4 hard) |
Fibre Profile/ Shape |
Round |
Round |
Round |
Rectangle |
Rectangle |
Size (diameter) |
Inches |
0.003 |
0.003 |
0.003 |
0.001 x 0.003 |
0.001 x 0.003 |
mm |
0.0762 |
0.0762 |
0.0762 |
0.0254 x 0.0762 |
0.0254 x 0.0762 |
Average Tensile Strength |
ksi |
174.0 |
333.6 |
145.0 |
304.6 |
145.0 |
GPa |
1.2 |
2.3 |
1.0 |
2.1 |
1.0 |
Average Elengation at Break (%) |
1.7 |
1.7 |
31.1 |
3.1 |
1.7 |
Average Tensile Modules |
ksi |
10,877.8 |
29,399.1 |
19,507.6 |
27,470.1 |
21,886.2 |
GPa |
75.0 |
202.7 |
134.5 |
189.4 |
150.9 |
In order to provide extra support and context for the use of this novel material, the ZEUS LCP fiber was compared to a number of non-metal materials that have been used in the construction of catheters such as nylon, polyether ether ketone (PEEK), polyethylene terephthalate, and Kevlar®. Interestingly, Kevlar® is a multifilament yarn while PET, PEEK, and nylon are monofilaments. It was observed that the ZEUS LCP monofilament outperformed all the monofilament fibers in terms of modulus and tensile strength while showing the least elongation at break. It also exhibited excellent tensile modulus to the Kevlar® multifilament, as shown in Table 2. Additionally, the LCP displayed the least shrinkage among all the monofilament fibers tested up to 483 °F. Overall, these comparisons demonstrate that the ZEUS LCP monofilament is a better alternative to practically all other non-metal fibers suggested for catheter braiding.
Table 2. Comparison of properties of the LCP monofilament fiber and several materials commonly used in catheter construction. Polymer data is from: http://www.matweb.com/, PET, PEEK, and Nylon 6 data were for unreinforced material. Range values are due to the range of grades tested. PET, PEEK, and Nylon 6 shrinkages were linearly measured at temperatures not exceeding 302 °F; LCP fiber shrinkage was linearly measured at 390 °F (199 °C) and at 482 °F (250 °C). Kevlar® 29 fiber was 1500 denier/1000 filaments/dtex 1670; data was taken from Du PONT. ksi = 1000 x psi; MPa = 106 x pascal; GPa = 109 x pascal; mm = millimeter.
|
Zeus LCP Fiber |
PET |
PEEK |
Nylon 6 |
Kevlar* 29 |
Average Tensile Strength |
ksi |
174.0 |
4.9 |
7.98 - 13.9 |
6.3 - 9.2 |
424 |
|
1.2 GPa |
33.9 MPa |
55.0 - 95.5 MPa |
45.0 - 67.7 MPa |
12.9 GPa |
Average Elengation at Break (%) |
1.7 |
84.3 |
19.3 - 35.0 |
76.3 |
3.6 |
Average Tensile Modules |
ksi |
10,877.8 |
424.0 |
499 - 612 |
245.0 - 331.0 |
10.2 |
test |
GPa |
75.0 |
2.9 |
3.4 - 4.2 |
1.7 - 2.28 |
70.5 |
Shrinkage (%) |
<0.01 |
0.5 - 1.1 |
1.2 |
1.4 |
<0.1 |
Manufacturability with respect to catheter construction is another benefit that the LCP fiber has over other braiding materials. Kevlar® is a multifilament yarn and hence it is prone to bird nesting or fraying when employed in braiding machines, while almost no fraying is shown by the LCP monofilament. Also, during the last stages of construction, Kevlar® shows some amount of flattening when it is covered with heat shrink to reflow the catheter jacket material (such as nylon or Pebax). This spreading out or flattening of the Kevlar® fibers partly obstructs the interstitial space between the braiding and prevents complete bonding of the jacket to the underlying liner (such as etched OD PTFE tubing) during reflow (Figure 10).
A troublesome issue with catheter construction is inadequate bonding. This can result in catheters with poor torsional and stiffness properties. Since the LCP fiber is a monofilament, it enables better penetration and bondability of the jacket to the underlying liner during the construction of catheter and is adequately strong to provide the required stiffness, torquability, and deflectability valued by clinicians.
.jpg)
Figure 10. Comparison of Zeus LCP monofilament fiber and Kevlar® as catheter braiding. (A ) Enlarged view of catheter braiding showing interstitial space between the braiding. Enlarged view of : (B) LCP monofilament, and (C) Kevlar® yarn with frayed fibrils. B and C are taken from 10x original images and are shown in the same scale. The Kevlar® shown is 200 dtex, and the LCP fiber is 0.003” diameter.
Conclusion
ZEUS’ LCP monofilament fiber represents an innovative offering as an LCP product and meets the pressing requirement in the medical sector: to produce a fully MRI-compatible catheter. The Zeus LCP fiber outperforms other polymer products such as common polyester and nylon in terms of tensile modulus (stiffness), strength, shrinkage, and elongation at least an order of magnitude. While the LCP monofilament is at least similar to the multifilament Kevlar® with respect to mechanical properties, it is different from the latter with its superior bondability of the liner and reflowed jacket covering during reflow. The LCP, as a substitute for stainless steel for catheter braiding, shows highly favorable characteristics: It maintains a degree of flexibility required for a deflectable catheter and provides considerable strength for pushability, stiffness, and torquability. With regard to stainless steel, the LCP monofilament acts as an amalgam between spring tempered (hard) stainless steel and annealed (soft) stainless steel, yet it is not metallic. The use of non-metal LCP reinforcement means that soft tissue visualization processes conducted along with catheterization can be achieved using non-radiological MRI. With this novel opportunity, clinicians and manufacturers alike have taken a renewed interest in this major step towards attaining a catheter that is fully compatible with MRI.
Download the Full White Paper Here
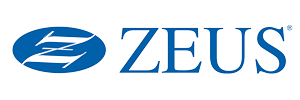
This information has been sourced, reviewed and adapted from materials provided by Zeus Industrial Products, Inc.
For more information on this source, please visit Zeus Industrial Products, Inc.