As topotactic transitions between comparable crystalline structures are a significant developing approach for the production of novel quantum elements, a topotactic reduction of single crystals with CaH2 as the reducing agent has been detailed in the latest research published in Science Advances.
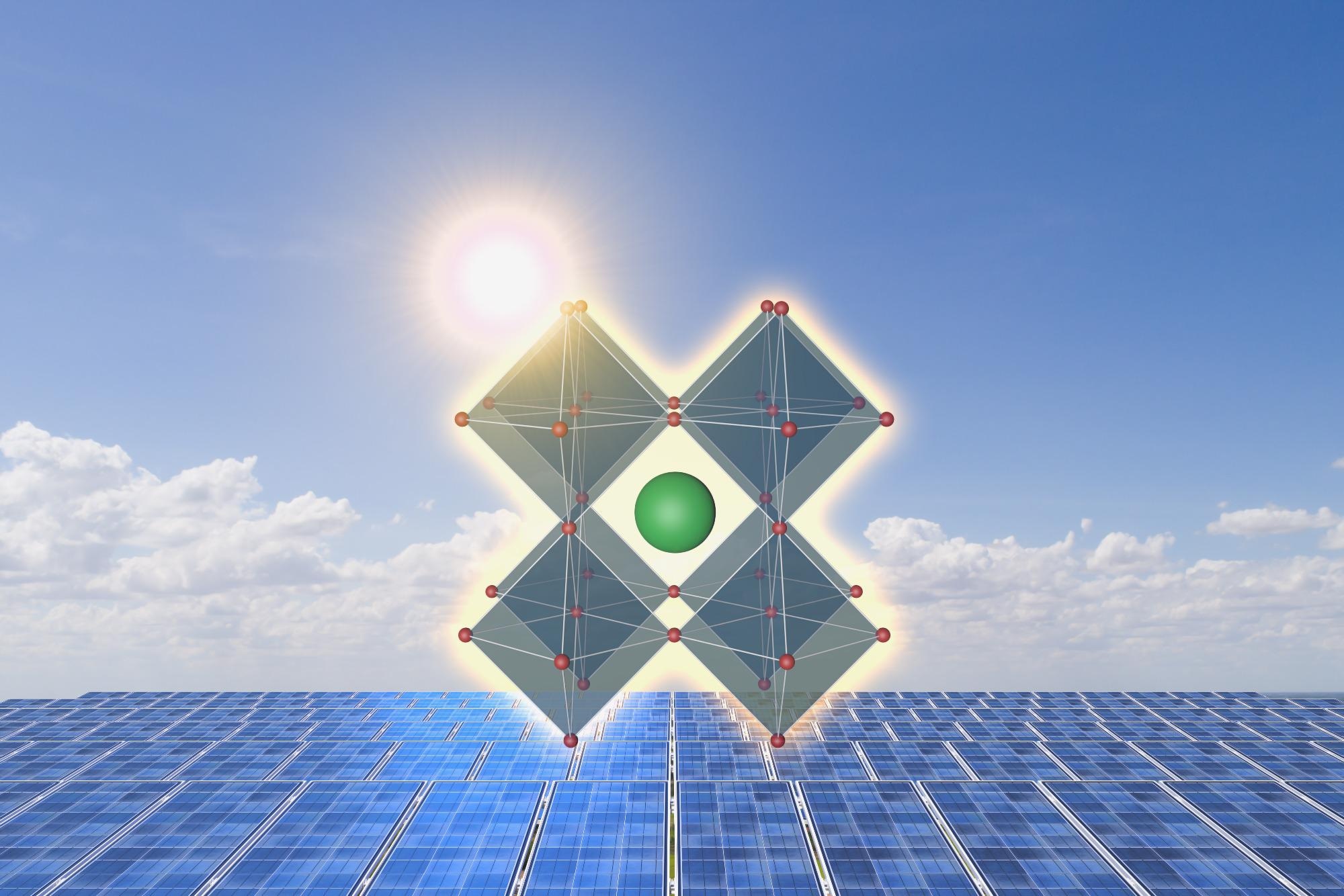
Study: Topotactic transformation of single crystals: From perovskite to infinite-layer nickelates. Image Credit: sutadimages/Shutterstock.com
Introduction to Perovskite
Perovskite is a substance with almost the same crystalline lattice as the mineral calcium titanium oxide, which was the first perovskite crystal found. Perovskite compounds, in general, have the molecular formula ABX3, where 'A' and 'B' are cations and X is an anion that links to both.
The lattice of perovskite (CaTiO3) is orthorhombic. Perovskite materials have been reported as being the most intriguing and economical minimal energy semiconductors for use in a wide range of photoelectric and optical technological applications. The finding of calcium titanate (CaTiO3) by a Russian mineralogist Perovski in 1839 was thought to be the genesis of perovskite, and minerals having the same sort of crystal structure as CaTiO3 were known as perovskite materials (structure).
Synthesis of perovskite nickelate crystals and topotactic reduction. (A) Schematic drawing of a hydraulic press with a Walker module. The uniaxial force (red arrows) is transferred to an isotropic pressure via a ceramic octahedron (brown) that is embedded in eight tungsten-carbide cubes. (B) Schematic of the cross section of the octahedron with a zirconia crucible inserted (yellow) filled with a Pt capsule (gray line). The salt flux and the nickelate precursor are depicted as white and gray areas in the capsule, respectively. During the growth process, a current is driven through a graphite heater (black line) leading to an external temperature gradient ∆T (red line). Crystals form at the top and bottom of the capsule (black cubes). (C) Externally applied pressure and temperature of the graphite heater plotted as a function of time. (D) SEM-SE image of an as-grown perovskite single crystal. (E) XRD map of the (h0l) planes of an as-grown perovskite single crystal. (F) Crystal structure of an as-grown perovskite crystal with space group R3¯c according to the refinement of the XRD data in (E). (G) SEM-SE image of a polycrystal after prolonged reduction with CaH2. Separation of domains can be recognized. (H) XRD map of a crystal reduced for a shorter time (see text). The inset shows a set of three reflections corresponding to three orthogonal domains with infinite-layer crystal structure. (I) Crystal structure of an infinite-layer crystal with space group P4/mmm according to the refinement of the XRD data in (H). Image Credit: Puphal, P. et al., Science Advances
Uses and Importance of Perovskite
Perovskite structures may include ions of varying size and charge, demonstrating remarkable compositional versatility. Because of its diverse and helpful features in photocatalytic, electrochromic, image storage, shifting, filtration, and subsurface sound waves signal conditioning technologies, perovskites have sparked a lot of interest in a variety of applications. Since it was revealed that perovskite solar cells are also extraordinarily effective at capturing photons of light and converting them into an electric charge, research focus on them has soared. A perovskite solar cell is a form of solar cell that uses a perovskite crystalline substance as the light-harvesting active material, most typically a hybrid organic-inorganic lead or tin halide-based material. Perovskite materials are often inexpensive and straightforward to make making them a viable option for various applications.
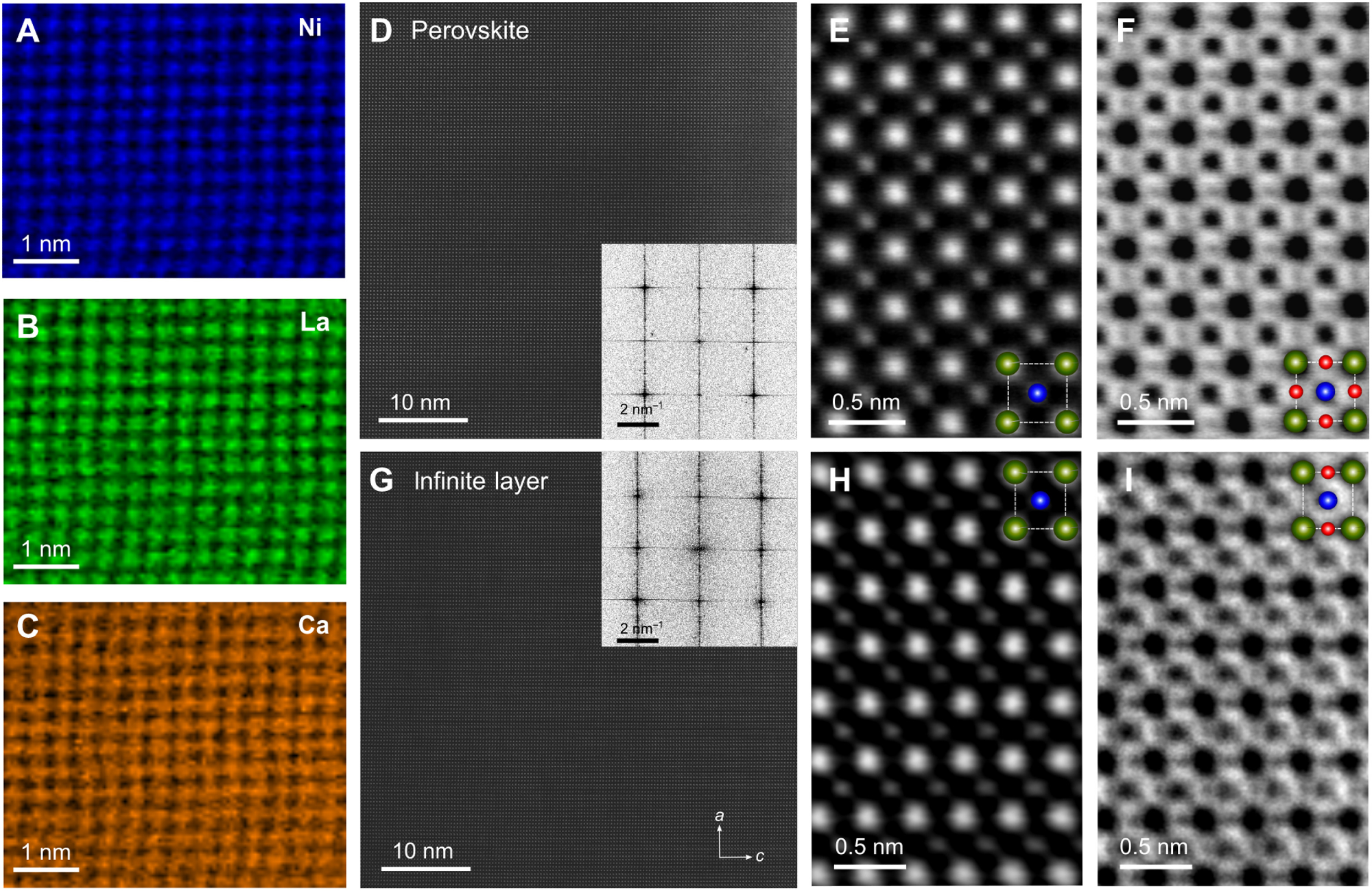
Topotactic transformation of the crystal structure. (A to C) Atomic-resolution STEM-EELS elemental maps of an as-grown perovskite crystal (x = 0.16) demonstrating the homogeneous distribution of Ni (A; blue), La (B; green), and Ca (C; orange) atoms. The maps were acquired simultaneously. (D and G) Low-magnification STEM-HAADF images of the perovskite and the reduced crystal, respectively, showing the absence of extended crystallographic defects. Insets correspond to the fast Fourier transformation of the HAADF images. (E and F) High-magnification STEM-HAADF and STEM-ABF images of the perovskite crystal. Both images were acquired from the same part of the STEM specimen. The superimposed cartoon indicates a pseudocubic unit cell with different elements highlighted according to their color in the elemental maps (A to C). Oxygen atoms can be identified specifically in the STEM-ABF image (F). (H and I) STEM-HAADF and STEM-ABF images of the reduced crystal, in analogy to (E) and (F). Image Credit: Puphal, P. et al., Science Advances
Limitations of Perovskite Material
Although Perovskite material has ideal properties yet several limitations and disadvantages are also a hurdle in its wide commercialization. The technology is still in its early stages, and the industry is wary of utilizing it to replace existing silicon PV technology. Other issues to be concerned about include degradation and stability. One important issue is that they have a short life expectancy when compared to silicon technology, its primary rival. The material is also not stable; lead in perovskite oxide readily leads to iodine volatility, and it decomposes easily when crystals are moist. If we utilize perovskite battery power, the seepage flow to the roof or in the soil is likely to disintegrate. As a result, further study is required to address these constraints and problems.
Transformation Process for Infinite-Layer Nickelates
Topotactic reductions of perovskite-related transition metals have paved the way for the development of whole new families of multifunctional, optoelectronic, and electromagnetic substances. The finding of superconductivity in infinite-layer nickelate thin films was a recent breakthrough in science. Single-crystalline specimens with at least micrometer-sized single dimensions are widely needed to reveal the inherent features of nickelates' infinite-layer phase. Furthermore, single crystals enable the use of complementary measuring methods and can demonstrate greater crystalline nature.
![Magnetic properties and electrical transport. (A) Magnetic susceptibility of an as-grown perovskite (black) and a reduced (red) crystal measured upon zero field cooling (ZFC, dotted lines) and field cooling (FC, solid lines) in a small external field of 0.03 T. (B) Susceptibility in a strong field of 7 T. The solid gray and orange lines are fits with a Curie-type law (see text). (C) Resistivity of a perovskite single crystal [x = 0.07(2)]. (D) Resistance of a reduced crystal [x = 0.08(2)] normalized to the room temperature value. The inset shows an SEM-SE image of a fragment of the infinite-layer crystal used in both the electrical transport and STEM measurements.](https://www.azom.com/images/news/ImageForNews_57715_16396578166594259.png)
Magnetic properties and electrical transport. (A) Magnetic susceptibility of an as-grown perovskite (black) and a reduced (red) crystal measured upon zero field cooling (ZFC, dotted lines) and field cooling (FC, solid lines) in a small external field of 0.03 T. (B) Susceptibility in a strong field of 7 T. The solid gray and orange lines are fits with a Curie-type law (see text). (C) Resistivity of a perovskite single crystal [x = 0.07(2)]. (D) Resistance of a reduced crystal [x = 0.08(2)] normalized to the room temperature value. The inset shows an SEM-SE image of a fragment of the infinite-layer crystal used in both the electrical transport and STEM measurements. Image Credit: Puphal, P. et al., Science Advances
Research Findings
Crystals with a typical size of 150 mm by 150 mm by 150 mm were obtained. The perovskite crystals were reduced to the infinite-layer phase La1−xCaxNiO2+d using CaH2. It was discovered that if the temperatures surpass a particular threshold, or if the retention durations are too long for the given Pt foil depth, the fluxes can dissolve too much of the Pt packet and the oxygen pressure is discharged, resulting in the disintegration of any developed specimen. The nickelate progressively dissolves at increased temperatures at 1300 °C, and transport growth begins. The results of energy-dispersive x-ray spectroscopy (EDS) and single-crystal x-ray diffraction (XRD) show that the Ca replacement level in the produced crystals is significantly lower than the nominal level of 20 atomic percent (at percent) predicted from the educts.
It was found that extending the two weeks by a few days did not result in noticeable modifications in crystals. Significantly longer decreasing durations were discovered to increase the fragility of the crystals.
In short, the transformation of perovskite into infinite-layer nickelates has paved the paths for various future applications. In addition to the extremely intrusive topotactic reduction, the fabrication of the precursor perovskite phase is a major obstacle in the production of infinite-layer nickelate crystals, although concentrating on a more research-oriented approach might result in a better knowledge of the unique process.
References
Puphal, P. et al., 2021. Topotactic transformation of single crystals: From perovskite to infinite-layer nickelates. Science Advances, 7(49). Available at: https://www.science.org/doi/10.1126/sciadv.abl8091
Disclaimer: The views expressed here are those of the author expressed in their private capacity and do not necessarily represent the views of AZoM.com Limited T/A AZoNetwork the owner and operator of this website. This disclaimer forms part of the Terms and conditions of use of this website.